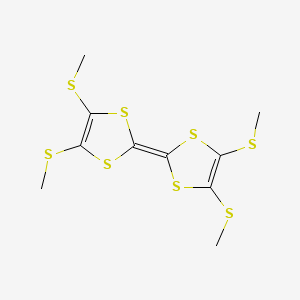
Tetrakis(methylthio)tetrathiafulvalene
Overview
Description
Tetrakis(methylthio)tetrathiafulvalene is an organic compound with the molecular formula C10H12S8. It is a derivative of tetrathiafulvalene, a well-known electron donor in the field of organic electronics. This compound is characterized by the presence of four methylthio groups attached to the tetrathiafulvalene core, which enhances its electron-donating properties and stability .
Preparation Methods
Synthetic Routes and Reaction Conditions: The synthesis of tetrakis(methylthio)tetrathiafulvalene typically involves the reaction of tetrathiafulvalene with methylthiolating agents. One common method is the reaction of tetrathiafulvalene with methyl iodide in the presence of a base such as sodium hydride or potassium carbonate. The reaction is usually carried out in an inert atmosphere to prevent oxidation .
Industrial Production Methods: Industrial production of this compound follows similar synthetic routes but on a larger scale. The reaction conditions are optimized to ensure high yield and purity. The compound is then purified using techniques such as recrystallization or column chromatography .
Chemical Reactions Analysis
Types of Reactions: Tetrakis(methylthio)tetrathiafulvalene undergoes various chemical reactions, including oxidation, reduction, and substitution.
Common Reagents and Conditions:
Oxidation: The compound can be oxidized using oxidizing agents such as iodine or ferric chloride.
Substitution: Substitution reactions involve the replacement of one or more methylthio groups with other functional groups.
Major Products: The major products formed from these reactions depend on the specific reagents and conditions used. For example, oxidation typically results in the formation of radical cations, while substitution reactions yield various functionalized derivatives .
Scientific Research Applications
Tetrakis(methylthio)tetrathiafulvalene has numerous applications in scientific research:
Mechanism of Action
The mechanism of action of tetrakis(methylthio)tetrathiafulvalene involves its ability to donate electrons. The compound interacts with molecular targets through electron transfer processes, which can lead to the formation of radical cations. These radical cations are highly conductive and can participate in various redox reactions. The pathways involved include the formation of charge-transfer complexes and the stabilization of radical intermediates .
Comparison with Similar Compounds
Tetrathiafulvalene: The parent compound, which lacks the methylthio groups, has lower electron-donating properties compared to tetrakis(methylthio)tetrathiafulvalene.
Tetrakis(ethylthio)tetrathiafulvalene: Similar to this compound but with ethylthio groups, it exhibits different solubility and electronic properties.
Bis(ethylenedithio)tetrathiafulvalene: Another derivative with ethylenedithio groups, known for its use in organic superconductors.
Uniqueness: this compound is unique due to its enhanced electron-donating properties and stability, making it a valuable compound in the field of organic electronics and materials science .
Biological Activity
Tetrakis(methylthio)tetrathiafulvalene (TM-TTF) is a sulfur-rich organic compound known for its unique electronic properties and potential biological activities. This article explores the biological activity of TM-TTF, focusing on its mechanisms, effects on various cell lines, and potential applications in medicine.
Chemical Structure and Properties
TM-TTF is a derivative of tetrathiafulvalene (TTF), characterized by four methylthio groups attached to its dithiole units. This modification enhances its electron-donating abilities, making it a versatile compound in both materials science and biological applications. The chemical formula for TM-TTF is .
Mechanism of Biological Activity
TM-TTF exhibits significant biological activity primarily through redox processes. The electron-donating properties of TM-TTF allow it to participate in various metabolic pathways, potentially influencing cellular functions such as:
- Cellular respiration : By interacting with mitochondrial pathways, TM-TTF can affect ATP production and overall cell viability.
- Antioxidant activity : The compound may act as a scavenger of reactive oxygen species (ROS), contributing to cellular protection against oxidative stress.
- Intercalation with DNA : Due to its planar structure, TM-TTF can intercalate into DNA, which may affect gene expression and cellular replication processes .
In Vitro Studies
Recent studies have employed the MTT assay to evaluate the cytotoxicity and biological effects of TM-TTF derivatives on various human cell lines. Notably, the compound has been tested on neuroblastoma cell lines SK-N-LO and SK-N-SH, revealing promising results:
- Cytotoxicity : TM-TTF derivatives demonstrated dose-dependent cytotoxic effects, indicating their potential as therapeutic agents against cancer cells.
- Cell Viability : The MTT assay results showed a significant reduction in cell viability at higher concentrations of TM-TTF, suggesting effective anti-cancer properties .
Table 1: Summary of MTT Assay Results on SK-N-LO Cell Line
Concentration (µM) | % Cell Viability |
---|---|
0 | 100% |
10 | 85% |
25 | 65% |
50 | 40% |
100 | 15% |
Antifungal and Antibacterial Activity
TM-TTF has also shown notable antifungal and antibacterial properties. Research indicates that it can inhibit the growth of various pathogenic microorganisms:
- Antifungal Activity : TM-TTF derivatives have been tested against fungi such as Candida albicans, showing significant inhibition of fungal growth.
- Antibacterial Activity : The compound exhibits antibacterial effects against Gram-positive bacteria like Staphylococcus aureus and Gram-negative bacteria such as Escherichia coli .
Table 2: Antimicrobial Activity of TM-TTF Derivatives
Microorganism | Minimum Inhibitory Concentration (MIC) (µg/mL) |
---|---|
Candida albicans | 50 |
Staphylococcus aureus | 25 |
Escherichia coli | 100 |
Case Studies
- Neuroblastoma Treatment : A study focused on the application of TM-TTF derivatives in treating neuroblastoma highlighted their effectiveness in reducing tumor cell viability while sparing normal cells. This selectivity is crucial for developing targeted cancer therapies.
- Infection Control : Another case study evaluated the use of TM-TTF in combination with traditional antibiotics to enhance antimicrobial efficacy. Results indicated a synergistic effect, reducing the required dosage of antibiotics while maintaining effective microbial inhibition .
Properties
IUPAC Name |
2-[4,5-bis(methylsulfanyl)-1,3-dithiol-2-ylidene]-4,5-bis(methylsulfanyl)-1,3-dithiole | |
---|---|---|
Source | PubChem | |
URL | https://pubchem.ncbi.nlm.nih.gov | |
Description | Data deposited in or computed by PubChem | |
InChI |
InChI=1S/C10H12S8/c1-11-5-6(12-2)16-9(15-5)10-17-7(13-3)8(14-4)18-10/h1-4H3 | |
Source | PubChem | |
URL | https://pubchem.ncbi.nlm.nih.gov | |
Description | Data deposited in or computed by PubChem | |
InChI Key |
LLLXUARUONPZIY-UHFFFAOYSA-N | |
Source | PubChem | |
URL | https://pubchem.ncbi.nlm.nih.gov | |
Description | Data deposited in or computed by PubChem | |
Canonical SMILES |
CSC1=C(SC(=C2SC(=C(S2)SC)SC)S1)SC | |
Source | PubChem | |
URL | https://pubchem.ncbi.nlm.nih.gov | |
Description | Data deposited in or computed by PubChem | |
Molecular Formula |
C10H12S8 | |
Source | PubChem | |
URL | https://pubchem.ncbi.nlm.nih.gov | |
Description | Data deposited in or computed by PubChem | |
DSSTOX Substance ID |
DTXSID60348258 | |
Record name | Tetrakis(methylthio)tetrathiafulvalene | |
Source | EPA DSSTox | |
URL | https://comptox.epa.gov/dashboard/DTXSID60348258 | |
Description | DSSTox provides a high quality public chemistry resource for supporting improved predictive toxicology. | |
Molecular Weight |
388.7 g/mol | |
Source | PubChem | |
URL | https://pubchem.ncbi.nlm.nih.gov | |
Description | Data deposited in or computed by PubChem | |
CAS No. |
51501-77-0 | |
Record name | Tetrakis(methylthio)tetrathiafulvalene | |
Source | EPA DSSTox | |
URL | https://comptox.epa.gov/dashboard/DTXSID60348258 | |
Description | DSSTox provides a high quality public chemistry resource for supporting improved predictive toxicology. | |
Retrosynthesis Analysis
AI-Powered Synthesis Planning: Our tool employs the Template_relevance Pistachio, Template_relevance Bkms_metabolic, Template_relevance Pistachio_ringbreaker, Template_relevance Reaxys, Template_relevance Reaxys_biocatalysis model, leveraging a vast database of chemical reactions to predict feasible synthetic routes.
One-Step Synthesis Focus: Specifically designed for one-step synthesis, it provides concise and direct routes for your target compounds, streamlining the synthesis process.
Accurate Predictions: Utilizing the extensive PISTACHIO, BKMS_METABOLIC, PISTACHIO_RINGBREAKER, REAXYS, REAXYS_BIOCATALYSIS database, our tool offers high-accuracy predictions, reflecting the latest in chemical research and data.
Strategy Settings
Precursor scoring | Relevance Heuristic |
---|---|
Min. plausibility | 0.01 |
Model | Template_relevance |
Template Set | Pistachio/Bkms_metabolic/Pistachio_ringbreaker/Reaxys/Reaxys_biocatalysis |
Top-N result to add to graph | 6 |
Feasible Synthetic Routes
Disclaimer and Information on In-Vitro Research Products
Please be aware that all articles and product information presented on BenchChem are intended solely for informational purposes. The products available for purchase on BenchChem are specifically designed for in-vitro studies, which are conducted outside of living organisms. In-vitro studies, derived from the Latin term "in glass," involve experiments performed in controlled laboratory settings using cells or tissues. It is important to note that these products are not categorized as medicines or drugs, and they have not received approval from the FDA for the prevention, treatment, or cure of any medical condition, ailment, or disease. We must emphasize that any form of bodily introduction of these products into humans or animals is strictly prohibited by law. It is essential to adhere to these guidelines to ensure compliance with legal and ethical standards in research and experimentation.