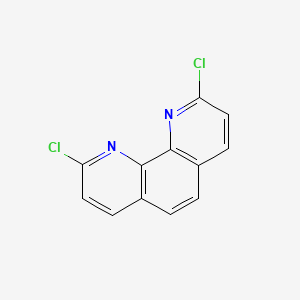
2,9-Dichloro-1,10-phenanthroline
Overview
Description
2,9-Dichloro-1,10-phenanthroline is an organic compound with the molecular formula C₁₂H₆Cl₂N₂. It is a derivative of 1,10-phenanthroline, where two chlorine atoms are substituted at the 2 and 9 positions. This compound is known for its planar structure and delocalized π-electrons in the aromatic fused-ring system .
Preparation Methods
Synthetic Routes and Reaction Conditions
2,9-Dichloro-1,10-phenanthroline can be synthesized through the chlorination of N,N′-annelated phenanthrolinediones. One such method involves the chlorination of 3,6,7,9-tetrahydro-5H-[1,4]diazepino[1,2,3,4-lmn][1,10]phenanthroline-3,9-dione . The reaction typically requires specific conditions to ensure the simultaneous introduction of two chlorine substituents to the non-substituted 1,10-phenanthroline.
Industrial Production Methods
While detailed industrial production methods are not widely documented, the synthesis generally involves similar chlorination reactions under controlled conditions to achieve high purity and yield.
Chemical Reactions Analysis
Types of Reactions
2,9-Dichloro-1,10-phenanthroline undergoes various chemical reactions, including:
Substitution Reactions: The chlorine atoms can be substituted with other functional groups.
Coordination Reactions: It can form complexes with metals, which are useful in catalysis and other applications.
Common Reagents and Conditions
Common reagents used in reactions with this compound include:
Metal Salts: For coordination reactions.
Nucleophiles: For substitution reactions.
Major Products
The major products formed from these reactions depend on the specific reagents and conditions used. For example, coordination with metal salts can lead to the formation of metal-phenanthroline complexes .
Scientific Research Applications
2,9-Dichloro-1,10-phenanthroline has a wide range of applications in scientific research:
Chemistry: Used as a ligand in coordination chemistry to form metal complexes.
Biology: Employed in studies involving DNA interactions and enzyme inhibition.
Medicine: Investigated for its potential therapeutic properties, including antimicrobial and anticancer activities.
Mechanism of Action
The mechanism by which 2,9-Dichloro-1,10-phenanthroline exerts its effects involves its ability to coordinate with metal ions. This coordination can influence various molecular targets and pathways, such as:
Enzyme Inhibition: By binding to metal ions in the active sites of enzymes, it can inhibit their activity.
DNA Interactions: It can intercalate with DNA, affecting its structure and function.
Comparison with Similar Compounds
Similar Compounds
1,10-Phenanthroline: The parent compound without chlorine substitutions.
4,7-Dichloro-1,10-phenanthroline: Another derivative with chlorine atoms at different positions.
2,9-Dimethyl-1,10-phenanthroline: A derivative with methyl groups instead of chlorine.
Uniqueness
2,9-Dichloro-1,10-phenanthroline is unique due to its specific substitution pattern, which influences its chemical reactivity and coordination properties. The presence of chlorine atoms enhances its ability to form stable complexes with metals, making it particularly useful in catalysis and other applications .
Properties
IUPAC Name |
2,9-dichloro-1,10-phenanthroline | |
---|---|---|
Source | PubChem | |
URL | https://pubchem.ncbi.nlm.nih.gov | |
Description | Data deposited in or computed by PubChem | |
InChI |
InChI=1S/C12H6Cl2N2/c13-9-5-3-7-1-2-8-4-6-10(14)16-12(8)11(7)15-9/h1-6H | |
Source | PubChem | |
URL | https://pubchem.ncbi.nlm.nih.gov | |
Description | Data deposited in or computed by PubChem | |
InChI Key |
DNKGIDURJINUOA-UHFFFAOYSA-N | |
Source | PubChem | |
URL | https://pubchem.ncbi.nlm.nih.gov | |
Description | Data deposited in or computed by PubChem | |
Canonical SMILES |
C1=CC2=C(C3=C1C=CC(=N3)Cl)N=C(C=C2)Cl | |
Source | PubChem | |
URL | https://pubchem.ncbi.nlm.nih.gov | |
Description | Data deposited in or computed by PubChem | |
Molecular Formula |
C12H6Cl2N2 | |
Source | PubChem | |
URL | https://pubchem.ncbi.nlm.nih.gov | |
Description | Data deposited in or computed by PubChem | |
DSSTOX Substance ID |
DTXSID00326671 | |
Record name | 2,9-DICHLORO-1,10-PHENANTHROLINE | |
Source | EPA DSSTox | |
URL | https://comptox.epa.gov/dashboard/DTXSID00326671 | |
Description | DSSTox provides a high quality public chemistry resource for supporting improved predictive toxicology. | |
Molecular Weight |
249.09 g/mol | |
Source | PubChem | |
URL | https://pubchem.ncbi.nlm.nih.gov | |
Description | Data deposited in or computed by PubChem | |
CAS No. |
29176-55-4 | |
Record name | 2,9-Dichloro-1,10-phenanthroline | |
Source | CAS Common Chemistry | |
URL | https://commonchemistry.cas.org/detail?cas_rn=29176-55-4 | |
Description | CAS Common Chemistry is an open community resource for accessing chemical information. Nearly 500,000 chemical substances from CAS REGISTRY cover areas of community interest, including common and frequently regulated chemicals, and those relevant to high school and undergraduate chemistry classes. This chemical information, curated by our expert scientists, is provided in alignment with our mission as a division of the American Chemical Society. | |
Explanation | The data from CAS Common Chemistry is provided under a CC-BY-NC 4.0 license, unless otherwise stated. | |
Record name | 2,9-DICHLORO-1,10-PHENANTHROLINE | |
Source | EPA DSSTox | |
URL | https://comptox.epa.gov/dashboard/DTXSID00326671 | |
Description | DSSTox provides a high quality public chemistry resource for supporting improved predictive toxicology. | |
Synthesis routes and methods
Procedure details
Retrosynthesis Analysis
AI-Powered Synthesis Planning: Our tool employs the Template_relevance Pistachio, Template_relevance Bkms_metabolic, Template_relevance Pistachio_ringbreaker, Template_relevance Reaxys, Template_relevance Reaxys_biocatalysis model, leveraging a vast database of chemical reactions to predict feasible synthetic routes.
One-Step Synthesis Focus: Specifically designed for one-step synthesis, it provides concise and direct routes for your target compounds, streamlining the synthesis process.
Accurate Predictions: Utilizing the extensive PISTACHIO, BKMS_METABOLIC, PISTACHIO_RINGBREAKER, REAXYS, REAXYS_BIOCATALYSIS database, our tool offers high-accuracy predictions, reflecting the latest in chemical research and data.
Strategy Settings
Precursor scoring | Relevance Heuristic |
---|---|
Min. plausibility | 0.01 |
Model | Template_relevance |
Template Set | Pistachio/Bkms_metabolic/Pistachio_ringbreaker/Reaxys/Reaxys_biocatalysis |
Top-N result to add to graph | 6 |
Feasible Synthetic Routes
Q1: What are the common synthetic routes to 2,9-Dichloro-1,10-phenanthroline?
A1: Two main synthetic routes exist. The conventional method involves a six-step process starting from 1,10-phenanthroline, resulting in a 44% yield of this compound [, ]. An alternative three-step synthesis utilizes 1,10-phenanthroline-N,N'-dioxide as a starting material [, ]. Researchers have also explored using N,N′-annelated phenanthrolinediones as precursors for a more efficient synthesis [, ].
Q2: What makes this compound a valuable precursor in organic synthesis?
A2: The two chlorine atoms in the 2,9-positions of this compound make it highly reactive. This allows for easy functionalization via nucleophilic substitution reactions, enabling the synthesis of diverse 1,10-phenanthroline derivatives. For instance, it can be used to create 2,9-diamino, 2,9-dialkoxy, and 2,9-diacetyl analogues [, , ].
Q3: How does the structure of this compound influence its reactivity?
A3: this compound exhibits a nearly planar structure with a slight deviation of the carbon atoms []. The C-N and C-C bond distances within the molecule suggest a delocalized π-electron system across the fused rings, contributing to its reactivity in substitution reactions.
Q4: Can this compound be used to synthesize macrocyclic compounds?
A4: Yes, this compound acts as a building block for macrocycles. Reacting it with 2-hydroxyethylhydrazine forms 2,9-di(N-2′-hydroxyethylhydrazino)-1,10-phenanthroline, which can further undergo template condensation with 2,6-diacetylpyridine to yield a macrocyclic complex []. Additionally, condensation with 2,9-diamino-1,10-phenanthroline produces a novel macrocyclic ligand in excellent yield [].
Q5: What is the significance of this compound in coordination chemistry?
A5: this compound is a valuable precursor for synthesizing various multidentate ligands. For example, it can be used to create a tetradentate ligand, 2,9-di-(2′-pyridyl)-1,10-phenanthroline, through a Stille coupling reaction with 2-(tri-n-butylstannyl)pyridine []. This tetradentate ligand readily forms complexes with Ru(II), showcasing its versatility in coordination chemistry.
Q6: How does this compound contribute to the synthesis of chiral concave 1,10-phenanthrolines?
A6: this compound plays a crucial role in synthesizing chiral concave 1,10-phenanthrolines []. These concave molecules are generated by attaching alkenyloxy-substituted aryl bridgeheads to this compound through Suzuki coupling or nucleophilic aromatic substitution, followed by ring-closing metathesis []. These chiral concave 1,10-phenanthrolines have shown promising results as ligands in copper(I)-catalyzed diastereoselective cyclopropanation reactions.
Q7: Are there alternative synthetic approaches to aryl-substituted 1,10-phenanthrolines?
A7: Yes, Suzuki coupling reactions utilizing this compound and substituted areneboronic acids provide a facile route to diverse mono- or diaryl-substituted 1,10-phenanthrolines []. This method allows for the efficient introduction of various substituents onto the 1,10-phenanthroline scaffold, expanding its potential applications.
Q8: How does this compound contribute to the development of fluorescent materials?
A8: this compound serves as a starting material for synthesizing aza[7]helicenes with high fluorescence quantum yields []. These helicenes, featuring a unique 6-5-6-6-6-5-6 skeleton, are synthesized through a two-step process involving double amination with aniline derivatives followed by an intramolecular double-NH/CH coupling mediated by a hypervalent iodine reagent.
Q9: Can this compound be used in analytical chemistry?
A9: Yes, incorporating this compound into carbon paste electrodes enhances the detection of silver ions []. This modification enables the electrode to chemically bind silver ions, leading to a significantly improved voltammetric response compared to unmodified electrodes.
Q10: What are the applications of this compound derivatives in material science?
A10: Derivatives like 2,2';9',2''-Ter[1,10]phenanthroline, synthesized from this compound, exhibit unique properties []. For instance, this ligand acts as a hexadentate with K+ and forms a trinuclear, helical complex with Cu(I), showcasing its potential in supramolecular chemistry and materials science.
Disclaimer and Information on In-Vitro Research Products
Please be aware that all articles and product information presented on BenchChem are intended solely for informational purposes. The products available for purchase on BenchChem are specifically designed for in-vitro studies, which are conducted outside of living organisms. In-vitro studies, derived from the Latin term "in glass," involve experiments performed in controlled laboratory settings using cells or tissues. It is important to note that these products are not categorized as medicines or drugs, and they have not received approval from the FDA for the prevention, treatment, or cure of any medical condition, ailment, or disease. We must emphasize that any form of bodily introduction of these products into humans or animals is strictly prohibited by law. It is essential to adhere to these guidelines to ensure compliance with legal and ethical standards in research and experimentation.