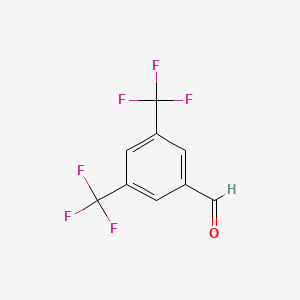
3,5-Bis(trifluoromethyl)benzaldehyde
Overview
Description
3,5-Bis(trifluoromethyl)benzaldehyde is a chemical compound with the molecular formula C9H4F6O and a molecular weight of 242.12 g/mol . It is characterized by the presence of two trifluoromethyl groups attached to a benzaldehyde core. This compound is a colorless liquid with a density of 1.469 g/mL at 25°C and a boiling point of 188.51°C . It is commonly used as an intermediate in the synthesis of various pharmaceuticals and agrochemicals.
Mechanism of Action
Biochemical Analysis
Biochemical Properties
It is known that the compound has high electronegativity and large steric hindrance . These properties could potentially influence its interactions with enzymes, proteins, and other biomolecules.
Cellular Effects
A derivative of this compound, 3,5-bis(trifluoromethyl)benzyl, has been used to modify a triazine-based covalent organic framework, which successfully suppressed the diffusion of polysulfides, leading to improved capacity and cyclic stability of lithium-sulfur batteries .
Temporal Effects in Laboratory Settings
It is known that the compound has a boiling point of 37 °C at 1.3 mmHg and a density of 1.469 g/mL at 25 °C .
Preparation Methods
Synthetic Routes and Reaction Conditions
3,5-Bis(trifluoromethyl)benzaldehyde can be synthesized through several methods. One common method involves the oxidation of 3,5-bis(trifluoromethyl)benzyl alcohol using oxidizing agents such as TEMPO (2,2,6,6-Tetramethylpiperidine 1-oxyl) and nitric acid in the presence of hydrochloric acid . The reaction is typically carried out in dichloromethane as a solvent at room temperature for about 10 hours .
Industrial Production Methods
In industrial settings, the production of this compound may involve the use of continuous flow reactors to ensure consistent quality and yield. The process often includes the use of advanced oxidation techniques and catalysts to enhance the efficiency of the reaction.
Chemical Reactions Analysis
Types of Reactions
3,5-Bis(trifluoromethyl)benzaldehyde undergoes various chemical reactions, including:
Oxidation: It can be oxidized to form 3,5-bis(trifluoromethyl)benzoic acid.
Reduction: It can be reduced to form 3,5-bis(trifluoromethyl)benzyl alcohol.
Substitution: It can undergo nucleophilic substitution reactions to form various derivatives.
Common Reagents and Conditions
Oxidation: Common oxidizing agents include TEMPO and nitric acid in the presence of hydrochloric acid.
Reduction: Reducing agents such as sodium borohydride or lithium aluminum hydride can be used.
Substitution: Nucleophiles such as amines or thiols can be used under basic conditions.
Major Products Formed
Oxidation: 3,5-Bis(trifluoromethyl)benzoic acid.
Reduction: 3,5-Bis(trifluoromethyl)benzyl alcohol.
Substitution: Various substituted benzaldehyde derivatives.
Scientific Research Applications
3,5-Bis(trifluoromethyl)benzaldehyde is widely used in scientific research due to its unique chemical properties. Some of its applications include:
Biology: It is used in the study of enzyme mechanisms and as a probe in biochemical assays.
Medicine: It serves as an intermediate in the synthesis of pharmaceuticals, particularly those containing trifluoromethyl groups, which are known to enhance the bioavailability and metabolic stability of drugs[][4].
Industry: It is used in the production of agrochemicals and specialty chemicals.
Comparison with Similar Compounds
3,5-Bis(trifluoromethyl)benzaldehyde can be compared with other similar compounds, such as:
3,4,5-Trifluorobenzaldehyde: Contains three fluorine atoms on the benzene ring.
2,3,4,5,6-Pentafluorobenzaldehyde: Contains five fluorine atoms on the benzene ring.
4-(Trifluoromethyl)benzaldehyde: Contains a single trifluoromethyl group on the benzene ring.
Uniqueness
The presence of two trifluoromethyl groups in this compound makes it unique compared to other fluorinated benzaldehydes. This structural feature imparts distinct electronic and steric properties, making it a valuable intermediate in the synthesis of various complex molecules .
Properties
IUPAC Name |
3,5-bis(trifluoromethyl)benzaldehyde | |
---|---|---|
Source | PubChem | |
URL | https://pubchem.ncbi.nlm.nih.gov | |
Description | Data deposited in or computed by PubChem | |
InChI |
InChI=1S/C9H4F6O/c10-8(11,12)6-1-5(4-16)2-7(3-6)9(13,14)15/h1-4H | |
Source | PubChem | |
URL | https://pubchem.ncbi.nlm.nih.gov | |
Description | Data deposited in or computed by PubChem | |
InChI Key |
LDWLIXZSDPXYDR-UHFFFAOYSA-N | |
Source | PubChem | |
URL | https://pubchem.ncbi.nlm.nih.gov | |
Description | Data deposited in or computed by PubChem | |
Canonical SMILES |
C1=C(C=C(C=C1C(F)(F)F)C(F)(F)F)C=O | |
Source | PubChem | |
URL | https://pubchem.ncbi.nlm.nih.gov | |
Description | Data deposited in or computed by PubChem | |
Molecular Formula |
C9H4F6O | |
Source | PubChem | |
URL | https://pubchem.ncbi.nlm.nih.gov | |
Description | Data deposited in or computed by PubChem | |
DSSTOX Substance ID |
DTXSID80193141 | |
Record name | 3,5-Bis(trifluoromethyl)benzaldehyde | |
Source | EPA DSSTox | |
URL | https://comptox.epa.gov/dashboard/DTXSID80193141 | |
Description | DSSTox provides a high quality public chemistry resource for supporting improved predictive toxicology. | |
Molecular Weight |
242.12 g/mol | |
Source | PubChem | |
URL | https://pubchem.ncbi.nlm.nih.gov | |
Description | Data deposited in or computed by PubChem | |
CAS No. |
401-95-6 | |
Record name | 3,5-Bis(trifluoromethyl)benzaldehyde | |
Source | CAS Common Chemistry | |
URL | https://commonchemistry.cas.org/detail?cas_rn=401-95-6 | |
Description | CAS Common Chemistry is an open community resource for accessing chemical information. Nearly 500,000 chemical substances from CAS REGISTRY cover areas of community interest, including common and frequently regulated chemicals, and those relevant to high school and undergraduate chemistry classes. This chemical information, curated by our expert scientists, is provided in alignment with our mission as a division of the American Chemical Society. | |
Explanation | The data from CAS Common Chemistry is provided under a CC-BY-NC 4.0 license, unless otherwise stated. | |
Record name | 3,5-Bis(trifluoromethyl)benzaldehyde | |
Source | ChemIDplus | |
URL | https://pubchem.ncbi.nlm.nih.gov/substance/?source=chemidplus&sourceid=0000401956 | |
Description | ChemIDplus is a free, web search system that provides access to the structure and nomenclature authority files used for the identification of chemical substances cited in National Library of Medicine (NLM) databases, including the TOXNET system. | |
Record name | 3,5-Bis(trifluoromethyl)benzaldehyde | |
Source | EPA DSSTox | |
URL | https://comptox.epa.gov/dashboard/DTXSID80193141 | |
Description | DSSTox provides a high quality public chemistry resource for supporting improved predictive toxicology. | |
Record name | 3,5-Bis(trifluoromethyl)benzaldehyde | |
Source | European Chemicals Agency (ECHA) | |
URL | https://echa.europa.eu/information-on-chemicals | |
Description | The European Chemicals Agency (ECHA) is an agency of the European Union which is the driving force among regulatory authorities in implementing the EU's groundbreaking chemicals legislation for the benefit of human health and the environment as well as for innovation and competitiveness. | |
Explanation | Use of the information, documents and data from the ECHA website is subject to the terms and conditions of this Legal Notice, and subject to other binding limitations provided for under applicable law, the information, documents and data made available on the ECHA website may be reproduced, distributed and/or used, totally or in part, for non-commercial purposes provided that ECHA is acknowledged as the source: "Source: European Chemicals Agency, http://echa.europa.eu/". Such acknowledgement must be included in each copy of the material. ECHA permits and encourages organisations and individuals to create links to the ECHA website under the following cumulative conditions: Links can only be made to webpages that provide a link to the Legal Notice page. | |
Synthesis routes and methods
Procedure details
Retrosynthesis Analysis
AI-Powered Synthesis Planning: Our tool employs the Template_relevance Pistachio, Template_relevance Bkms_metabolic, Template_relevance Pistachio_ringbreaker, Template_relevance Reaxys, Template_relevance Reaxys_biocatalysis model, leveraging a vast database of chemical reactions to predict feasible synthetic routes.
One-Step Synthesis Focus: Specifically designed for one-step synthesis, it provides concise and direct routes for your target compounds, streamlining the synthesis process.
Accurate Predictions: Utilizing the extensive PISTACHIO, BKMS_METABOLIC, PISTACHIO_RINGBREAKER, REAXYS, REAXYS_BIOCATALYSIS database, our tool offers high-accuracy predictions, reflecting the latest in chemical research and data.
Strategy Settings
Precursor scoring | Relevance Heuristic |
---|---|
Min. plausibility | 0.01 |
Model | Template_relevance |
Template Set | Pistachio/Bkms_metabolic/Pistachio_ringbreaker/Reaxys/Reaxys_biocatalysis |
Top-N result to add to graph | 6 |
Feasible Synthetic Routes
Disclaimer and Information on In-Vitro Research Products
Please be aware that all articles and product information presented on BenchChem are intended solely for informational purposes. The products available for purchase on BenchChem are specifically designed for in-vitro studies, which are conducted outside of living organisms. In-vitro studies, derived from the Latin term "in glass," involve experiments performed in controlled laboratory settings using cells or tissues. It is important to note that these products are not categorized as medicines or drugs, and they have not received approval from the FDA for the prevention, treatment, or cure of any medical condition, ailment, or disease. We must emphasize that any form of bodily introduction of these products into humans or animals is strictly prohibited by law. It is essential to adhere to these guidelines to ensure compliance with legal and ethical standards in research and experimentation.