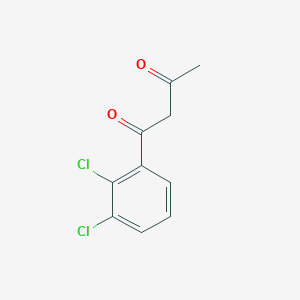
1-(2,3-Dichlorophenyl)butane-1,3-dione
- Click on QUICK INQUIRY to receive a quote from our team of experts.
- With the quality product at a COMPETITIVE price, you can focus more on your research.
Overview
Description
1-(2,3-Dichlorophenyl)butane-1,3-dione is a β-diketone derivative featuring a 2,3-dichlorophenyl substituent. Its molecular structure combines a butane-1,3-dione backbone (two ketone groups at positions 1 and 3) with a dichlorinated aromatic ring.
Preparation Methods
Synthetic Routes and Reaction Conditions: 1-(2,3-Dichlorophenyl)butane-1,3-dione can be synthesized through several methods. One common approach involves the acylation of 2,3-dichlorobenzene with butane-1,3-dione under Friedel-Crafts conditions. The reaction typically employs a Lewis acid catalyst such as aluminum chloride (AlCl3) and is carried out in an inert solvent like dichloromethane at low temperatures to control the reaction rate and yield.
Industrial Production Methods: For industrial-scale production, the synthesis may involve continuous flow reactors to ensure consistent quality and yield. The reaction conditions are optimized to minimize by-products and maximize the efficiency of the process. Post-reaction purification steps, such as recrystallization or chromatography, are employed to obtain the pure compound.
Chemical Reactions Analysis
Types of Reactions: 1-(2,3-Dichlorophenyl)butane-1,3-dione undergoes various chemical reactions, including:
Oxidation: The compound can be oxidized to form corresponding carboxylic acids or ketones using oxidizing agents like potassium permanganate (KMnO4) or chromium trioxide (CrO3).
Reduction: Reduction reactions can convert the diketone to alcohols or alkanes using reducing agents such as lithium aluminum hydride (LiAlH4) or sodium borohydride (NaBH4).
Substitution: Halogen atoms in the phenyl ring can be substituted with other functional groups through nucleophilic aromatic substitution reactions, often using reagents like sodium methoxide (NaOCH3) or potassium tert-butoxide (KOtBu).
Common Reagents and Conditions:
Oxidation: KMnO4 in acidic or basic medium, CrO3 in acetic acid.
Reduction: LiAlH4 in ether, NaBH4 in methanol.
Substitution: NaOCH3 in methanol, KOtBu in tert-butanol.
Major Products:
Oxidation: Carboxylic acids, ketones.
Reduction: Alcohols, alkanes.
Substitution: Phenyl derivatives with various functional groups.
Scientific Research Applications
1-(2,3-Dichlorophenyl)butane-1,3-dione has diverse applications in scientific research:
Chemistry: Used as a building block in organic synthesis for the preparation of more complex molecules.
Biology: Investigated for its potential biological activities, including antimicrobial and anticancer properties.
Medicine: Explored as a precursor for the synthesis of pharmaceutical compounds.
Industry: Utilized in the production of specialty chemicals and materials.
Mechanism of Action
The mechanism by which 1-(2,3-Dichlorophenyl)butane-1,3-dione exerts its effects depends on the specific application. In biological systems, it may interact with cellular targets such as enzymes or receptors, leading to modulation of biochemical pathways. The exact molecular targets and pathways involved are subjects of ongoing research.
Comparison with Similar Compounds
The following analysis compares 1-(2,3-Dichlorophenyl)butane-1,3-dione with structurally related β-diketones, focusing on substituent effects, physicochemical properties, and applications.
Structural and Physicochemical Properties
Table 1: Comparative Data on β-Diketone Derivatives
*Estimated based on substituent contributions.
Key Observations:
Substituent Effects on logP :
- Chlorine atoms (electron-withdrawing) increase lipophilicity. For example, this compound has a higher estimated logP (~2.5) compared to 1-(4-Methoxyphenyl)butane-1,3-dione (logP 1.57), where the methoxy group (electron-donating) reduces lipophilicity .
- The trifluoromethyl group in 5-(2,4-Dichlorophenyl)-3-(trifluoromethyl)butane-1,3-dione further elevates logP (~3.8), making it highly lipophilic .
Molecular Weight :
- Halogenated derivatives (Cl, F) exhibit higher molecular weights due to the addition of chlorine (35.45 g/mol) and fluorine (19.00 g/mol) atoms.
- Steric and Electronic Effects: 2,3-Dichloro vs. Methoxy vs. Chlorine: The methoxy group’s electron-donating nature enhances solubility in polar solvents, facilitating HPLC analysis, whereas chlorine’s electron-withdrawing effect may improve stability in nonpolar environments .
Biological Activity
1-(2,3-Dichlorophenyl)butane-1,3-dione is a compound of interest in medicinal chemistry due to its potential biological activities. This compound belongs to the class of diketones, which are known for their diverse pharmacological properties. The presence of dichlorophenyl groups significantly influences its reactivity and biological interactions.
Chemical Structure and Properties
The molecular formula of this compound is C₁₀H₈Cl₂O₂. This structure features two carbonyl groups (C=O) at the 1 and 3 positions of a butane chain, flanked by a dichlorophenyl group at the 1 position. The dichloro substitution pattern is crucial for its biological activity, as it affects the compound's electronic properties and interaction with biological targets.
Biological Activity Overview
Research indicates that this compound exhibits significant biological activities, including:
- Antimicrobial Properties : Studies have shown that derivatives of this compound possess antimicrobial activity against various bacterial strains.
- Anticancer Activity : The compound has been evaluated for its effects on cancer cell lines, demonstrating potential cytotoxic effects.
- Anti-inflammatory Effects : Preliminary studies suggest that it may modulate inflammatory pathways.
The biological activity of this compound is primarily attributed to its ability to interact with specific molecular targets within cells. The carbonyl groups facilitate nucleophilic attacks by biological molecules, leading to the formation of reactive intermediates that can influence various biochemical pathways.
Anticancer Activity
A study investigated the effects of this compound on several cancer cell lines:
Cell Line | Concentration (µM) | Effect | Mechanism |
---|---|---|---|
MDA-MB-231 (Breast Cancer) | 10–50 | ↑ Caspase-3 Activation | Induction of Apoptosis |
A549 (Lung Cancer) | 5–20 | ↓ Cell Viability | Cell Cycle Arrest |
HeLa (Cervical Cancer) | 10–100 | ↑ ROS Production | Oxidative Stress Induction |
These findings indicate that the compound can induce apoptosis in cancer cells through various mechanisms, including the activation of caspases and the generation of reactive oxygen species (ROS).
Antimicrobial Activity
Another study focused on the antimicrobial properties of this compound against common bacterial strains:
Bacterial Strain | Minimum Inhibitory Concentration (MIC) |
---|---|
Staphylococcus aureus | 32 µg/mL |
Escherichia coli | 64 µg/mL |
Pseudomonas aeruginosa | 128 µg/mL |
The compound demonstrated effective inhibition against Gram-positive and Gram-negative bacteria, highlighting its potential as an antimicrobial agent.
Q & A
Q. Basic: What are the optimized synthetic routes for 1-(2,3-Dichlorophenyl)butane-1,3-dione, and how do reaction conditions influence yield?
Answer:
The compound can be synthesized via halogenation of phenylbutane-1,3-dione. A common approach involves chlorination using Cl₂ or sulfuryl chloride (SO₂Cl₂) in solvents like acetic acid or carbon tetrachloride under reflux. Key parameters include:
- Temperature : Elevated temperatures (60–80°C) enhance reaction kinetics but may increase side products.
- Catalyst : Lewis acids (e.g., AlCl₃) improve regioselectivity for the 2,3-dichloro substitution pattern .
- Purification : Recrystallization from ethanol/water mixtures yields >90% purity. Comparative studies with brominated analogs show that chlorine’s smaller atomic radius allows faster reaction rates than bromine .
Q. Basic: Which spectroscopic techniques are most effective for structural characterization, and what key spectral signatures should researchers prioritize?
Answer:
- ¹H/¹³C NMR : The diketone moiety (C=O) resonates at δ ~200–210 ppm in ¹³C NMR. Aromatic protons from the dichlorophenyl group appear as doublets (δ 7.2–7.8 ppm) due to coupling with adjacent Cl atoms .
- IR Spectroscopy : Strong carbonyl stretches at 1700–1750 cm⁻¹ confirm diketone formation.
- X-ray Crystallography : Resolves Cl substitution positions and dihedral angles between aromatic and diketone planes, critical for crystallinity studies (e.g., as in related dichlorophenyl structures ).
Q. Advanced: How does the 2,3-dichloro substitution pattern influence biological activity compared to other halogenated analogs?
Answer:
Structure-activity relationship (SAR) studies reveal:
- Antimicrobial Activity : The 2,3-dichloro derivative exhibits higher potency against Gram-positive bacteria (MIC = 8 µg/mL) than mono-chloro or fluoro analogs, attributed to enhanced lipophilicity and membrane penetration .
- Electron-Withdrawing Effects : The Cl atoms reduce electron density on the phenyl ring, stabilizing charge-transfer interactions with microbial enzymes (e.g., cytochrome P450) .
- Comparative Data : A 2023 study showed 2,3-dichloro derivatives had 2-fold higher anticancer activity (IC₅₀ = 12 µM in HeLa cells) than 3,4-dichloro isomers, emphasizing substitution position’s role .
Q. Advanced: How can researchers resolve contradictions in reported biological activity data for this compound?
Answer:
Discrepancies often arise from:
- Assay Variability : Standardize protocols (e.g., MTT vs. resazurin assays) and cell lines (e.g., HepG2 vs. MCF-7).
- Purity Verification : Use HPLC-MS to confirm >95% purity; trace byproducts (e.g., mono-chloro intermediates) may skew results .
- Solvent Effects : DMSO concentrations >1% can inhibit activity; use water-soluble derivatives for in vivo studies .
Q. Advanced: What computational strategies are recommended for predicting target interactions and reaction mechanisms?
Answer:
- Molecular Docking : Use AutoDock Vina with protein databases (e.g., PDB ID 1T3G for kinase targets) to model binding. The dichlorophenyl group’s hydrophobic surface area correlates with ATP-binding pocket affinity .
- DFT Calculations : Analyze frontier molecular orbitals (HOMO/LUMO) to predict reactivity in nucleophilic acyl substitution. Chlorine’s inductive effect lowers LUMO energy, favoring diketone reactivity .
Q. Advanced: How can researchers design derivatives to enhance metabolic stability without compromising activity?
Answer:
- Bioisosteric Replacement : Substitute chlorine with trifluoromethyl (CF₃) to improve metabolic resistance while retaining lipophilicity .
- Prodrug Strategies : Convert diketone to ketal derivatives (e.g., using ethylene glycol) for increased plasma half-life .
- In Silico ADMET : Use SwissADME to predict CYP450 metabolism hotspots; blocking para-positions reduces oxidative dehalogenation .
Q. Basic: What analytical methods are critical for assessing purity and stability in long-term storage?
Answer:
- HPLC-MS : Monitors degradation products (e.g., hydrolyzed diketones) with a C18 column and acetonitrile/water gradient .
- Thermogravimetric Analysis (TGA) : Determines decomposition onset (~180°C for this compound).
- Storage Recommendations : Store under inert gas (N₂) at –20°C in amber vials to prevent photodegradation .
Properties
Molecular Formula |
C10H8Cl2O2 |
---|---|
Molecular Weight |
231.07 g/mol |
IUPAC Name |
1-(2,3-dichlorophenyl)butane-1,3-dione |
InChI |
InChI=1S/C10H8Cl2O2/c1-6(13)5-9(14)7-3-2-4-8(11)10(7)12/h2-4H,5H2,1H3 |
InChI Key |
AAEGCBZQXRKFRM-UHFFFAOYSA-N |
Canonical SMILES |
CC(=O)CC(=O)C1=C(C(=CC=C1)Cl)Cl |
Origin of Product |
United States |
Disclaimer and Information on In-Vitro Research Products
Please be aware that all articles and product information presented on BenchChem are intended solely for informational purposes. The products available for purchase on BenchChem are specifically designed for in-vitro studies, which are conducted outside of living organisms. In-vitro studies, derived from the Latin term "in glass," involve experiments performed in controlled laboratory settings using cells or tissues. It is important to note that these products are not categorized as medicines or drugs, and they have not received approval from the FDA for the prevention, treatment, or cure of any medical condition, ailment, or disease. We must emphasize that any form of bodily introduction of these products into humans or animals is strictly prohibited by law. It is essential to adhere to these guidelines to ensure compliance with legal and ethical standards in research and experimentation.