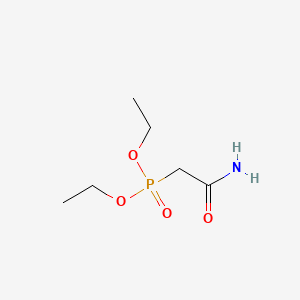
Diethyl (2-amino-2-oxoethyl)phosphonate
Overview
Description
Diethyl (2-amino-2-oxoethyl)phosphonate is an organophosphorus compound with the molecular formula C6H14NO4P. It is known for its applications in various fields, including organic synthesis and medicinal chemistry. The compound is characterized by the presence of a phosphonate group attached to a 2-amino-2-oxoethyl moiety, making it a versatile intermediate in chemical reactions.
Preparation Methods
Synthetic Routes and Reaction Conditions
Diethyl (2-amino-2-oxoethyl)phosphonate can be synthesized through several methods. One common approach involves the reaction of 2-chloroacetamide with triethyl phosphite. The reaction typically proceeds under mild conditions, with the use of a base such as sodium hydride to facilitate the formation of the phosphonate ester .
Industrial Production Methods
In industrial settings, the production of this compound often involves large-scale batch reactions. The process includes the careful control of reaction parameters such as temperature, pressure, and reactant concentrations to ensure high yield and purity of the final product .
Chemical Reactions Analysis
Types of Reactions
Diethyl (2-amino-2-oxoethyl)phosphonate undergoes various chemical reactions, including:
Oxidation: The compound can be oxidized to form corresponding phosphonic acids.
Reduction: Reduction reactions can convert the oxo group to a hydroxyl group.
Substitution: The amino group can participate in nucleophilic substitution reactions, leading to the formation of various derivatives.
Common Reagents and Conditions
Oxidation: Common oxidizing agents include hydrogen peroxide and potassium permanganate.
Reduction: Reducing agents such as lithium aluminum hydride are often used.
Substitution: Nucleophiles like amines and alcohols can react with the amino group under basic conditions.
Major Products
The major products formed from these reactions include phosphonic acids, hydroxyl derivatives, and various substituted phosphonates .
Scientific Research Applications
Diethyl (2-amino-2-oxoethyl)phosphonate has a wide range of applications in scientific research:
Chemistry: It is used as a building block in the synthesis of more complex organophosphorus compounds.
Medicine: Research is ongoing into its potential use in drug development, particularly for its ability to interact with biological targets.
Industry: It is used in the production of flame retardants, plasticizers, and other industrial chemicals.
Mechanism of Action
The mechanism by which diethyl (2-amino-2-oxoethyl)phosphonate exerts its effects involves its interaction with specific molecular targets. For example, it has been shown to bind selectively to one enantiomer of the enzyme carboxylic ester hydrolase, inhibiting its activity. This stereoselective binding is crucial for its effectiveness as an enzyme inhibitor .
Comparison with Similar Compounds
Similar Compounds
Diethyl (2-oxoethyl)phosphonate: Similar in structure but lacks the amino group, making it less versatile in certain reactions.
Diethyl (2-(dimethylamino)-2-oxoethyl)phosphonate: Contains a dimethylamino group instead of an amino group, which can affect its reactivity and applications.
Uniqueness
Diethyl (2-amino-2-oxoethyl)phosphonate is unique due to its combination of an amino group and a phosphonate ester, which allows it to participate in a wide range of chemical reactions and makes it a valuable intermediate in organic synthesis .
Biological Activity
Diethyl (2-amino-2-oxoethyl)phosphonate, a phosphonate compound, has garnered attention in scientific research for its diverse biological activities, particularly its potential as an enzyme inhibitor and antimicrobial agent. This article delves into its biological properties, mechanisms of action, and relevant case studies.
Chemical Structure and Properties
This compound is characterized by the presence of amino and oxo functional groups, which enhance its reactivity and biological activity. It is a colorless, water-soluble compound that exhibits slightly acidic properties. The phosphonate group plays a crucial role in its chemical behavior, allowing it to participate in various reactions such as hydrolysis and nucleophilic substitution.
Biological Activity
1. Enzyme Inhibition
This compound has been studied for its ability to inhibit carboxylic ester hydrolases, which are enzymes involved in the hydrolysis of esters. This inhibition can disrupt metabolic pathways in bacteria, making it a candidate for developing new antimicrobial agents.
2. Antimicrobial Properties
Research indicates that this compound can inhibit bacterial growth by binding to enzymes critical for bacterial metabolism. Specifically, it targets carboxylic acid enzymes, which are essential for various metabolic processes in bacteria. The compound's ability to form stable complexes with metal ions further enhances its potential as an antimicrobial agent.
The mechanism by which this compound exerts its biological effects primarily involves:
- Enzyme Binding : The compound binds to specific enzymes, inhibiting their activity and disrupting metabolic pathways.
- Nucleophilic Substitution : The amino group allows for nucleophilic substitution reactions, leading to the formation of various derivatives with potentially different biological activities.
Case Study 1: Antimicrobial Activity
In a study examining the antimicrobial properties of this compound, researchers found that it effectively inhibited the growth of several bacterial strains. The compound was tested against common pathogens, demonstrating significant inhibition comparable to established antibiotics. This study highlights its potential utility in developing new antimicrobial therapies.
Case Study 2: Enzyme Inhibition
Another research effort focused on the enzyme inhibitory effects of this compound on carboxylic ester hydrolases. The results indicated that the compound could serve as a potent inhibitor, with implications for drug development aimed at targeting specific enzymatic pathways involved in disease processes .
Research Findings and Data Tables
Parameter | Value |
---|---|
Molecular Formula | CHNOP |
Solubility | Water-soluble |
pH | Slightly acidic |
Inhibition Concentration (IC50) | Varies by target enzyme |
Key Findings from Literature
- Antimicrobial Efficacy : Demonstrated significant growth inhibition against multiple bacterial strains.
- Enzyme Inhibition : Effective against carboxylic ester hydrolases with varying IC50 values depending on the specific enzyme target.
Properties
IUPAC Name |
2-diethoxyphosphorylacetamide | |
---|---|---|
Source | PubChem | |
URL | https://pubchem.ncbi.nlm.nih.gov | |
Description | Data deposited in or computed by PubChem | |
InChI |
InChI=1S/C6H14NO4P/c1-3-10-12(9,11-4-2)5-6(7)8/h3-5H2,1-2H3,(H2,7,8) | |
Source | PubChem | |
URL | https://pubchem.ncbi.nlm.nih.gov | |
Description | Data deposited in or computed by PubChem | |
InChI Key |
QPVCUQOSWAXEOQ-UHFFFAOYSA-N | |
Source | PubChem | |
URL | https://pubchem.ncbi.nlm.nih.gov | |
Description | Data deposited in or computed by PubChem | |
Canonical SMILES |
CCOP(=O)(CC(=O)N)OCC | |
Source | PubChem | |
URL | https://pubchem.ncbi.nlm.nih.gov | |
Description | Data deposited in or computed by PubChem | |
Molecular Formula |
C6H14NO4P | |
Source | PubChem | |
URL | https://pubchem.ncbi.nlm.nih.gov | |
Description | Data deposited in or computed by PubChem | |
DSSTOX Substance ID |
DTXSID60280088 | |
Record name | Diethyl (2-amino-2-oxoethyl)phosphonate | |
Source | EPA DSSTox | |
URL | https://comptox.epa.gov/dashboard/DTXSID60280088 | |
Description | DSSTox provides a high quality public chemistry resource for supporting improved predictive toxicology. | |
Molecular Weight |
195.15 g/mol | |
Source | PubChem | |
URL | https://pubchem.ncbi.nlm.nih.gov | |
Description | Data deposited in or computed by PubChem | |
CAS No. |
5464-68-6 | |
Record name | 5464-68-6 | |
Source | DTP/NCI | |
URL | https://dtp.cancer.gov/dtpstandard/servlet/dwindex?searchtype=NSC&outputformat=html&searchlist=15422 | |
Description | The NCI Development Therapeutics Program (DTP) provides services and resources to the academic and private-sector research communities worldwide to facilitate the discovery and development of new cancer therapeutic agents. | |
Explanation | Unless otherwise indicated, all text within NCI products is free of copyright and may be reused without our permission. Credit the National Cancer Institute as the source. | |
Record name | Diethyl (2-amino-2-oxoethyl)phosphonate | |
Source | EPA DSSTox | |
URL | https://comptox.epa.gov/dashboard/DTXSID60280088 | |
Description | DSSTox provides a high quality public chemistry resource for supporting improved predictive toxicology. | |
Record name | 5464-68-6 | |
Source | European Chemicals Agency (ECHA) | |
URL | https://echa.europa.eu/information-on-chemicals | |
Description | The European Chemicals Agency (ECHA) is an agency of the European Union which is the driving force among regulatory authorities in implementing the EU's groundbreaking chemicals legislation for the benefit of human health and the environment as well as for innovation and competitiveness. | |
Explanation | Use of the information, documents and data from the ECHA website is subject to the terms and conditions of this Legal Notice, and subject to other binding limitations provided for under applicable law, the information, documents and data made available on the ECHA website may be reproduced, distributed and/or used, totally or in part, for non-commercial purposes provided that ECHA is acknowledged as the source: "Source: European Chemicals Agency, http://echa.europa.eu/". Such acknowledgement must be included in each copy of the material. ECHA permits and encourages organisations and individuals to create links to the ECHA website under the following cumulative conditions: Links can only be made to webpages that provide a link to the Legal Notice page. | |
Synthesis routes and methods
Procedure details
Retrosynthesis Analysis
AI-Powered Synthesis Planning: Our tool employs the Template_relevance Pistachio, Template_relevance Bkms_metabolic, Template_relevance Pistachio_ringbreaker, Template_relevance Reaxys, Template_relevance Reaxys_biocatalysis model, leveraging a vast database of chemical reactions to predict feasible synthetic routes.
One-Step Synthesis Focus: Specifically designed for one-step synthesis, it provides concise and direct routes for your target compounds, streamlining the synthesis process.
Accurate Predictions: Utilizing the extensive PISTACHIO, BKMS_METABOLIC, PISTACHIO_RINGBREAKER, REAXYS, REAXYS_BIOCATALYSIS database, our tool offers high-accuracy predictions, reflecting the latest in chemical research and data.
Strategy Settings
Precursor scoring | Relevance Heuristic |
---|---|
Min. plausibility | 0.01 |
Model | Template_relevance |
Template Set | Pistachio/Bkms_metabolic/Pistachio_ringbreaker/Reaxys/Reaxys_biocatalysis |
Top-N result to add to graph | 6 |
Feasible Synthetic Routes
Disclaimer and Information on In-Vitro Research Products
Please be aware that all articles and product information presented on BenchChem are intended solely for informational purposes. The products available for purchase on BenchChem are specifically designed for in-vitro studies, which are conducted outside of living organisms. In-vitro studies, derived from the Latin term "in glass," involve experiments performed in controlled laboratory settings using cells or tissues. It is important to note that these products are not categorized as medicines or drugs, and they have not received approval from the FDA for the prevention, treatment, or cure of any medical condition, ailment, or disease. We must emphasize that any form of bodily introduction of these products into humans or animals is strictly prohibited by law. It is essential to adhere to these guidelines to ensure compliance with legal and ethical standards in research and experimentation.