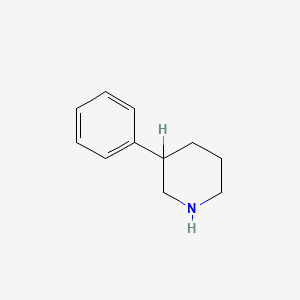
3-Phenylpiperidine
Overview
Description
3-Phenylpiperidine is an organic compound with the molecular formula C11H15N It consists of a piperidine ring substituted with a phenyl group at the third position
Mechanism of Action
Target of Action
3-Phenylpiperidine, a synthetic derivative of the phenylpiperidine class, primarily targets the central nervous system (CNS) μ-opioid receptors . These receptors play a crucial role in pain perception and response .
Mode of Action
This compound acts as an agonist at the μ-opioid receptors, leading to the inhibition of ascending pain pathways, altering pain perception, and resulting in CNS depression . It also has local anesthetic properties due to its interaction with sodium channels .
Biochemical Pathways
The primary biochemical pathway affected by this compound is the opioid signaling pathway. By acting as an agonist at the μ-opioid receptors, it inhibits the transmission of pain signals, thereby reducing the perception of pain .
Pharmacokinetics
This compound, like other phenylpiperidine derivatives, is quickly hydrolyzed in the liver to pethidinic acid and is also demethylated to norpethidine . Norpethidine is an active metabolite with analgesic activity and a potency twice that of this compound itself . Norpethidine has a longer elimination half-life and can accumulate in patients with renal disease, potentially leading to seizures .
Result of Action
The primary molecular effect of this compound is the activation of μ-opioid receptors, leading to altered pain perception and CNS depression . On a cellular level, this results in reduced transmission of pain signals, thereby providing analgesic effects .
Action Environment
The action, efficacy, and stability of this compound can be influenced by various environmental factors. For instance, the presence of other drugs can affect its metabolism and excretion, potentially leading to increased toxicity or reduced efficacy . Additionally, factors such as the patient’s age, liver function, and renal function can also influence the drug’s pharmacokinetics and overall effect .
Biochemical Analysis
Biochemical Properties
3-Phenylpiperidine plays a notable role in biochemical reactions, particularly in the context of its interactions with enzymes, proteins, and other biomolecules. It has been observed to interact with opioid receptors, specifically the μ-opioid receptor, where it acts as an agonist . This interaction is crucial for its analgesic properties. Additionally, this compound can inhibit the reuptake of neurotransmitters such as norepinephrine and serotonin, thereby influencing neurotransmission . These interactions highlight the compound’s potential in modulating pain and mood disorders.
Cellular Effects
The effects of this compound on various cell types and cellular processes are profound. It influences cell function by modulating cell signaling pathways, gene expression, and cellular metabolism. For instance, its interaction with opioid receptors can lead to the activation of G-protein coupled receptor signaling pathways, resulting in the inhibition of adenylate cyclase activity and a subsequent decrease in cyclic AMP levels . This modulation affects various downstream signaling pathways, ultimately influencing cellular responses such as pain perception and mood regulation.
Molecular Mechanism
At the molecular level, this compound exerts its effects through specific binding interactions with biomolecules. Its primary mechanism of action involves binding to the μ-opioid receptor, leading to receptor activation and subsequent intracellular signaling cascades . This binding inhibits adenylate cyclase, reduces cyclic AMP levels, and activates potassium channels while inhibiting calcium channels, resulting in hyperpolarization of neurons and reduced neuronal excitability . Additionally, this compound’s inhibition of norepinephrine and serotonin reuptake further contributes to its pharmacological effects .
Temporal Effects in Laboratory Settings
In laboratory settings, the effects of this compound have been observed to change over time. The compound exhibits stability under standard laboratory conditions, but its degradation can occur under extreme conditions such as high temperatures or acidic environments . Long-term studies have shown that prolonged exposure to this compound can lead to adaptive changes in cellular function, including receptor desensitization and downregulation . These temporal effects are crucial for understanding the compound’s long-term pharmacological implications.
Dosage Effects in Animal Models
The effects of this compound vary with different dosages in animal models. At low doses, the compound exhibits analgesic properties with minimal adverse effects . At higher doses, it can induce toxic effects such as respiratory depression, sedation, and potential neurotoxicity . These dosage-dependent effects highlight the importance of careful dose optimization in therapeutic applications to minimize adverse outcomes.
Metabolic Pathways
This compound is involved in several metabolic pathways, primarily in the liver. It undergoes hepatic metabolism through cytochrome P450 enzymes, particularly CYP2D6 and CYP3A4 . The primary metabolic pathways include N-dealkylation and hydroxylation, leading to the formation of various metabolites . These metabolic processes are essential for the compound’s clearance from the body and can influence its pharmacokinetic profile.
Transport and Distribution
Within cells and tissues, this compound is transported and distributed through passive diffusion and active transport mechanisms . It can interact with transporters such as P-glycoprotein, which influences its distribution across cellular membranes . The compound’s lipophilic nature facilitates its accumulation in lipid-rich tissues, including the brain, contributing to its central nervous system effects .
Subcellular Localization
The subcellular localization of this compound is primarily within the cytoplasm and cellular membranes . Its activity is influenced by its localization, as it needs to reach specific receptors and enzymes to exert its effects. Post-translational modifications and targeting signals can direct this compound to specific cellular compartments, enhancing its functional specificity .
Preparation Methods
Synthetic Routes and Reaction Conditions: 3-Phenylpiperidine can be synthesized through several methods. One common approach involves the reduction of 3-phenylpyridine using hydrogen in the presence of a catalyst such as palladium on carbon. Another method includes the cyclization of N-phenyl-1,3-diaminopropane under acidic conditions.
Industrial Production Methods: On an industrial scale, this compound is often produced via catalytic hydrogenation of 3-phenylpyridine. This process typically involves high pressure and temperature conditions to achieve efficient conversion rates. The use of robust catalysts like palladium or platinum is crucial for the reaction’s success.
Chemical Reactions Analysis
Types of Reactions: 3-Phenylpiperidine undergoes various chemical reactions, including:
Oxidation: It can be oxidized to form corresponding N-oxides or other oxidized derivatives.
Reduction: The compound can be reduced further to form more saturated derivatives.
Substitution: Electrophilic aromatic substitution reactions can occur on the phenyl ring, leading to various substituted phenylpiperidines.
Common Reagents and Conditions:
Oxidation: Reagents like hydrogen peroxide or peracids are commonly used.
Reduction: Catalysts such as palladium on carbon or lithium aluminum hydride are employed.
Substitution: Halogens, nitrating agents, and sulfonating agents are typical reagents used under controlled conditions.
Major Products:
Oxidation: N-oxides and hydroxylated derivatives.
Reduction: More saturated piperidine derivatives.
Substitution: Halogenated, nitrated, or sulfonated phenylpiperidines.
Scientific Research Applications
3-Phenylpiperidine has diverse applications in scientific research:
Chemistry: It serves as a building block in the synthesis of more complex organic molecules.
Biology: The compound is used in the study of neurotransmitter systems due to its structural similarity to certain bioactive molecules.
Medicine: It is investigated for its potential use in developing pharmaceuticals, particularly in the field of analgesics and antipsychotics.
Industry: this compound is used in the production of specialty chemicals and as an intermediate in the synthesis of agrochemicals and dyes.
Comparison with Similar Compounds
4-Phenylpiperidine: Known for its use in opioid analgesics.
2-Phenylpiperidine: Less common but studied for its unique pharmacological properties.
N-Phenylpiperidine: Used in various synthetic applications.
Uniqueness: 3-Phenylpiperidine is unique due to its specific substitution pattern, which imparts distinct chemical and biological properties. Its position on the piperidine ring influences its reactivity and interaction with biological targets, making it a valuable compound in medicinal chemistry.
By understanding the synthesis, reactions, applications, and mechanisms of this compound, researchers can better exploit its potential in various scientific and industrial fields.
Properties
IUPAC Name |
3-phenylpiperidine | |
---|---|---|
Source | PubChem | |
URL | https://pubchem.ncbi.nlm.nih.gov | |
Description | Data deposited in or computed by PubChem | |
InChI |
InChI=1S/C11H15N/c1-2-5-10(6-3-1)11-7-4-8-12-9-11/h1-3,5-6,11-12H,4,7-9H2 | |
Source | PubChem | |
URL | https://pubchem.ncbi.nlm.nih.gov | |
Description | Data deposited in or computed by PubChem | |
InChI Key |
NZYBILDYPCVNMU-UHFFFAOYSA-N | |
Source | PubChem | |
URL | https://pubchem.ncbi.nlm.nih.gov | |
Description | Data deposited in or computed by PubChem | |
Canonical SMILES |
C1CC(CNC1)C2=CC=CC=C2 | |
Source | PubChem | |
URL | https://pubchem.ncbi.nlm.nih.gov | |
Description | Data deposited in or computed by PubChem | |
Molecular Formula |
C11H15N | |
Source | PubChem | |
URL | https://pubchem.ncbi.nlm.nih.gov | |
Description | Data deposited in or computed by PubChem | |
DSSTOX Substance ID |
DTXSID30871048 | |
Record name | 3-Phenylpiperidine | |
Source | EPA DSSTox | |
URL | https://comptox.epa.gov/dashboard/DTXSID30871048 | |
Description | DSSTox provides a high quality public chemistry resource for supporting improved predictive toxicology. | |
Molecular Weight |
161.24 g/mol | |
Source | PubChem | |
URL | https://pubchem.ncbi.nlm.nih.gov | |
Description | Data deposited in or computed by PubChem | |
CAS No. |
3973-62-4 | |
Record name | 3-Phenylpiperidine | |
Source | CAS Common Chemistry | |
URL | https://commonchemistry.cas.org/detail?cas_rn=3973-62-4 | |
Description | CAS Common Chemistry is an open community resource for accessing chemical information. Nearly 500,000 chemical substances from CAS REGISTRY cover areas of community interest, including common and frequently regulated chemicals, and those relevant to high school and undergraduate chemistry classes. This chemical information, curated by our expert scientists, is provided in alignment with our mission as a division of the American Chemical Society. | |
Explanation | The data from CAS Common Chemistry is provided under a CC-BY-NC 4.0 license, unless otherwise stated. | |
Record name | 3-Phenylpiperidine | |
Source | ChemIDplus | |
URL | https://pubchem.ncbi.nlm.nih.gov/substance/?source=chemidplus&sourceid=0003973624 | |
Description | ChemIDplus is a free, web search system that provides access to the structure and nomenclature authority files used for the identification of chemical substances cited in National Library of Medicine (NLM) databases, including the TOXNET system. | |
Record name | 3-Phenylpiperidine | |
Source | EPA DSSTox | |
URL | https://comptox.epa.gov/dashboard/DTXSID30871048 | |
Description | DSSTox provides a high quality public chemistry resource for supporting improved predictive toxicology. | |
Record name | 3-phenylpiperidine | |
Source | European Chemicals Agency (ECHA) | |
URL | https://echa.europa.eu/substance-information/-/substanceinfo/100.021.458 | |
Description | The European Chemicals Agency (ECHA) is an agency of the European Union which is the driving force among regulatory authorities in implementing the EU's groundbreaking chemicals legislation for the benefit of human health and the environment as well as for innovation and competitiveness. | |
Explanation | Use of the information, documents and data from the ECHA website is subject to the terms and conditions of this Legal Notice, and subject to other binding limitations provided for under applicable law, the information, documents and data made available on the ECHA website may be reproduced, distributed and/or used, totally or in part, for non-commercial purposes provided that ECHA is acknowledged as the source: "Source: European Chemicals Agency, http://echa.europa.eu/". Such acknowledgement must be included in each copy of the material. ECHA permits and encourages organisations and individuals to create links to the ECHA website under the following cumulative conditions: Links can only be made to webpages that provide a link to the Legal Notice page. | |
Synthesis routes and methods I
Procedure details
Synthesis routes and methods II
Procedure details
Retrosynthesis Analysis
AI-Powered Synthesis Planning: Our tool employs the Template_relevance Pistachio, Template_relevance Bkms_metabolic, Template_relevance Pistachio_ringbreaker, Template_relevance Reaxys, Template_relevance Reaxys_biocatalysis model, leveraging a vast database of chemical reactions to predict feasible synthetic routes.
One-Step Synthesis Focus: Specifically designed for one-step synthesis, it provides concise and direct routes for your target compounds, streamlining the synthesis process.
Accurate Predictions: Utilizing the extensive PISTACHIO, BKMS_METABOLIC, PISTACHIO_RINGBREAKER, REAXYS, REAXYS_BIOCATALYSIS database, our tool offers high-accuracy predictions, reflecting the latest in chemical research and data.
Strategy Settings
Precursor scoring | Relevance Heuristic |
---|---|
Min. plausibility | 0.01 |
Model | Template_relevance |
Template Set | Pistachio/Bkms_metabolic/Pistachio_ringbreaker/Reaxys/Reaxys_biocatalysis |
Top-N result to add to graph | 6 |
Feasible Synthetic Routes
Q1: What are the primary biological targets of 3-Phenylpiperidine derivatives?
A1: this compound derivatives exhibit binding affinity for a range of biological targets, most notably sigma receptors (both σ1 and σ2 subtypes) [, ] and dopamine receptors (including D2 and D4 subtypes) [, , ]. They have also been investigated for their interactions with other targets like the β-catenin/B-cell lymphoma 9 (BCL9) protein-protein interaction [].
Q2: How does the interaction of 3-Phenylpiperidines with sigma receptors translate to their observed effects?
A2: While the precise mechanisms are still under investigation, binding to sigma receptors, particularly the σ1 subtype, has been linked to various pharmacological effects, including modulation of dopamine neurotransmission and potential therapeutic benefits in conditions like Parkinson's disease [, ].
Q3: Can you elaborate on the downstream effects of this compound binding to dopamine receptors?
A3: Depending on the specific subtype targeted and the intrinsic activity of the compound (agonist, antagonist, partial agonist), interactions with dopamine receptors can influence dopamine signaling pathways involved in motor control, reward, and cognition [, , ]. For instance, some 3-phenylpiperidines act as dopamine autoreceptor antagonists, potentially increasing dopamine release [].
Q4: What is the molecular formula and molecular weight of this compound?
A4: The molecular formula of this compound is C11H15N, and its molecular weight is 161.24 g/mol.
Q5: Is there any spectroscopic data available for characterizing this compound derivatives?
A5: Yes, researchers utilize various spectroscopic techniques, including NMR (Nuclear Magnetic Resonance) [], to analyze the structure and conformation of this compound derivatives. These analyses help determine the spatial arrangement of atoms and their influence on biological activity.
Q6: What is the significance of the aromatic hydroxyl group in this compound derivatives, particularly in relation to sigma receptor binding?
A7: Research suggests that while the aromatic hydroxyl group is a prominent feature in many sigma receptor ligands, its contribution to binding affinity in 3-Phenylpiperidines appears to be minimal []. This finding challenges previous assumptions and highlights the importance of systematic SAR studies.
Q7: How does the stereochemistry of 3-Phenylpiperidines impact their interaction with biological targets?
A8: Stereochemistry plays a crucial role in determining the activity and selectivity of this compound derivatives. For example, dextrorotatory isomers often display a higher affinity for σ1 receptors compared to their levorotatory counterparts [].
Q8: Can you provide an example of how conformational restriction in 3-Phenylpiperidines influences their activity?
A9: The synthesis of 1-phenyl-3-azabicyclo(3.1.0)hexanes, which are conformationally restricted analogs of 3-Phenylpiperidines, revealed that such restriction does not necessarily hinder sigma receptor affinity []. In fact, certain restricted analogs displayed enhanced selectivity for the σ1 receptor subtype, highlighting the potential of conformational control in drug design.
Q9: Are there any reported challenges related to the stability of this compound derivatives?
A10: While the provided research doesn't explicitly detail stability issues with this compound itself, the development of analogs like aminoglutethimide [] involved addressing metabolic instability and the formation of inactive metabolites. This emphasizes the importance of considering stability and metabolic liabilities during drug development.
Q10: Has the metabolic fate of this compound derivatives been investigated?
A11: Yes, studies have examined the metabolism of 3-Phenylpiperidines, specifically focusing on (S,S)-3-[3-(Methylsulfonyl)phenyl]-1-propylpiperidine hydrochloride [(-)-OSU6162]. Findings indicate that this compound undergoes N-dealkylation primarily mediated by CYP2D6, a cytochrome P450 enzyme, in human liver microsomes [].
Q11: Have this compound derivatives shown promise in preclinical models of disease?
A12: Research on a series of this compound derivatives targeting the β-catenin/BCL9 interaction demonstrated potent anti-colorectal cancer activity in vitro and in vivo []. These compounds suppressed Wnt signaling and tumor growth, highlighting their potential as anticancer agents.
Disclaimer and Information on In-Vitro Research Products
Please be aware that all articles and product information presented on BenchChem are intended solely for informational purposes. The products available for purchase on BenchChem are specifically designed for in-vitro studies, which are conducted outside of living organisms. In-vitro studies, derived from the Latin term "in glass," involve experiments performed in controlled laboratory settings using cells or tissues. It is important to note that these products are not categorized as medicines or drugs, and they have not received approval from the FDA for the prevention, treatment, or cure of any medical condition, ailment, or disease. We must emphasize that any form of bodily introduction of these products into humans or animals is strictly prohibited by law. It is essential to adhere to these guidelines to ensure compliance with legal and ethical standards in research and experimentation.