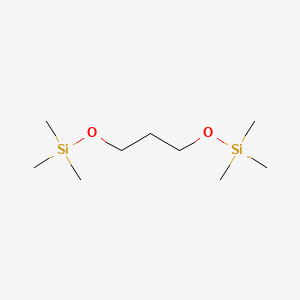
3,7-Dioxa-2,8-disilanonane, 2,2,8,8-tetramethyl-
Overview
Description
Tetrakis(di-tert-butylmethylsilyl)distannene and its Anion Radical
The synthesis of Tetrakis(di-tert-butylmethylsilyl)distannene was achieved through a coupling reaction involving tBu2MeSiNa and SnCl2-diox in THF, resulting in the formation of dark-green crystals. The molecular structure analysis revealed the presence of the shortest Sn=Sn double bond recorded among acyclic distannenes, with a length of 2.6683(10) Å. The geometry around the tin atoms was found to be almost planar, and the Sn=Sn double bond was highly twisted. Chemical reactions analysis indicated that the distannene did not dissociate into stannylenes in either solid state or solution, as evidenced by the production of 1,2-dichlorodistannane upon reaction with CCl4. Furthermore, the one-electron reduction of the distannene with potassium yielded a stable distannene anion radical, which was characterized by X-ray crystallography and ESR spectroscopy, marking it as a stable ion radical of the heavy alkene analogues .
Functional Polysiloxanes and Reactions with Amino Compounds
The study on functional polysiloxanes involved the reaction of 1,3-bis(3-glycidoxypropyl)-1,1,3,3-tetramethyldisiloxane with aromatic amino compounds, specifically aniline and p-aminobenzoic acid. The research focused on the influence of reaction conditions on the structure of the resulting compounds. Although the specific details of the physical and chemical properties of the synthesized organofunctional disiloxanes were not provided, the study implies that the reaction conditions play a significant role in determining the structure of the compounds formed .
Digermene and Disilene Chemistry
The unexpected reaction of tetrakis[di-tert-butyl(methyl)silyl]disilagermirenes with GeCl2.dioxane led to the quantitative formation of a novel compound, trans-1,2-dichloro-1,2,3,4-tetrakis[di-tert-butyl(methyl)silyl]-3Delta-1,2,3,4-disiladigermetene. This compound is notable for being the first cyclotetrametallene containing two different heavier group 14 elements and the first digermene incorporated into a four-membered ring. Additionally, a compound with a Ge=Sn double bond was synthesized through a similar reaction with SnCl2.dioxane. The molecular structure analysis of these compounds, particularly the folded four-membered ring skeleton and the long Ge=Ge double bond, was determined by X-ray crystallography. The study also discussed the structural peculiarities and the potential mechanism of formation for these compounds .
Scientific Research Applications
Synthesis and Chemical Reactions
- 1,2-Bis(1-diazo-2-oxoalkyl)-1,1,2,2-tetramethyl-disilanes, which include variants of 3,7-dioxa-2,8-disilanonane, have been synthesized and used in studies exploring UV light or transition metal catalysts induced decomposition, leading to rearranged, carbene-derived products (Maas & Fronda, 1990).
- Crystal structure analyses have been performed on various 1,3-dioxa-2-silacycloalkanes, providing insights into their molecular architecture and potential reactions with water and other substances (Hanson, Mcculloch, & Mcinnes, 1986).
Organosilicon Compounds
- Research on 1,1,2,2-tetramethyl-3,4,5,6-tetraphenyl-1,2-disila-3,4-cyclohexadiene, related to 3,7-dioxa-2,8-disilanonane, has been conducted, particularly focusing on its reactivity with singlet oxygen and the oxidation of the Si-Si bond (Nakadaira & Sakurai, 1973).
- Studies on the synthesis of difunctional organooxasilacycloalkanes have been performed, involving compounds like 2,8-disilanonane and its derivatives, to understand their chemical properties and potential applications (Chizhova et al., 2000).
Silicon-Based Drugs and Odorants
- Development of silicon-based drugs and odorants, using building blocks such as 4,4,5,5-tetramethyl-2-(3,5,5,8,8-pentamethyl-5,8-disila-5,6,7,8-tetrahydro-2-naphthyl)-1,3,2-dioxaborolane, demonstrates the potential pharmaceutical and sensory applications of these silicon-based compounds (Büttner, Nätscher, Burschka, & Tacke, 2007).
Polymer Science and Material Engineering
- In polymer science, 3,7-dioxa-2,8-disilanonane derivatives are used in epoxy curing systems for advancedelectronic packaging. These compounds can effectively lower the viscosity of epoxy systems and improve their mechanical properties (Li & Xie, 2009).
- The synthesis of polysiloxanes from systems involving tetraethoxysilane and ethyl alcohol has been explored, highlighting the formation of linear oligosiloxanes and the potential for creating novel siloxane materials (Sugahara et al., 1992).
Antimalarial Activity
- Research on methyl-substituted dispiro-1,2,4,5-tetraoxanes, which are structurally related to 3,7-dioxa-2,8-disilanonane, has revealed insights into antimalarial activity, underscoring the importance of structural features for medicinal applications (Mccullough et al., 2000).
properties
IUPAC Name |
trimethyl(3-trimethylsilyloxypropoxy)silane | |
---|---|---|
Source | PubChem | |
URL | https://pubchem.ncbi.nlm.nih.gov | |
Description | Data deposited in or computed by PubChem | |
InChI |
InChI=1S/C9H24O2Si2/c1-12(2,3)10-8-7-9-11-13(4,5)6/h7-9H2,1-6H3 | |
Source | PubChem | |
URL | https://pubchem.ncbi.nlm.nih.gov | |
Description | Data deposited in or computed by PubChem | |
InChI Key |
DWHYMKOUICVFHL-UHFFFAOYSA-N | |
Source | PubChem | |
URL | https://pubchem.ncbi.nlm.nih.gov | |
Description | Data deposited in or computed by PubChem | |
Canonical SMILES |
C[Si](C)(C)OCCCO[Si](C)(C)C | |
Source | PubChem | |
URL | https://pubchem.ncbi.nlm.nih.gov | |
Description | Data deposited in or computed by PubChem | |
Molecular Formula |
C9H24O2Si2 | |
Source | PubChem | |
URL | https://pubchem.ncbi.nlm.nih.gov | |
Description | Data deposited in or computed by PubChem | |
DSSTOX Substance ID |
DTXSID50170560 | |
Record name | 3,7-Dioxa-2,8-disilanonane, 2,2,8,8-tetramethyl- | |
Source | EPA DSSTox | |
URL | https://comptox.epa.gov/dashboard/DTXSID50170560 | |
Description | DSSTox provides a high quality public chemistry resource for supporting improved predictive toxicology. | |
Molecular Weight |
220.46 g/mol | |
Source | PubChem | |
URL | https://pubchem.ncbi.nlm.nih.gov | |
Description | Data deposited in or computed by PubChem | |
Product Name |
3,7-Dioxa-2,8-disilanonane, 2,2,8,8-tetramethyl- | |
CAS RN |
17887-80-8 | |
Record name | 3,7-Dioxa-2,8-disilanonane, 2,2,8,8-tetramethyl- | |
Source | ChemIDplus | |
URL | https://pubchem.ncbi.nlm.nih.gov/substance/?source=chemidplus&sourceid=0017887808 | |
Description | ChemIDplus is a free, web search system that provides access to the structure and nomenclature authority files used for the identification of chemical substances cited in National Library of Medicine (NLM) databases, including the TOXNET system. | |
Record name | 3,7-Dioxa-2,8-disilanonane, 2,2,8,8-tetramethyl- | |
Source | EPA DSSTox | |
URL | https://comptox.epa.gov/dashboard/DTXSID50170560 | |
Description | DSSTox provides a high quality public chemistry resource for supporting improved predictive toxicology. | |
Record name | 1,3-Bis(trimethylsilyloxy)propane | |
Source | European Chemicals Agency (ECHA) | |
URL | https://echa.europa.eu/information-on-chemicals | |
Description | The European Chemicals Agency (ECHA) is an agency of the European Union which is the driving force among regulatory authorities in implementing the EU's groundbreaking chemicals legislation for the benefit of human health and the environment as well as for innovation and competitiveness. | |
Explanation | Use of the information, documents and data from the ECHA website is subject to the terms and conditions of this Legal Notice, and subject to other binding limitations provided for under applicable law, the information, documents and data made available on the ECHA website may be reproduced, distributed and/or used, totally or in part, for non-commercial purposes provided that ECHA is acknowledged as the source: "Source: European Chemicals Agency, http://echa.europa.eu/". Such acknowledgement must be included in each copy of the material. ECHA permits and encourages organisations and individuals to create links to the ECHA website under the following cumulative conditions: Links can only be made to webpages that provide a link to the Legal Notice page. | |
Retrosynthesis Analysis
AI-Powered Synthesis Planning: Our tool employs the Template_relevance Pistachio, Template_relevance Bkms_metabolic, Template_relevance Pistachio_ringbreaker, Template_relevance Reaxys, Template_relevance Reaxys_biocatalysis model, leveraging a vast database of chemical reactions to predict feasible synthetic routes.
One-Step Synthesis Focus: Specifically designed for one-step synthesis, it provides concise and direct routes for your target compounds, streamlining the synthesis process.
Accurate Predictions: Utilizing the extensive PISTACHIO, BKMS_METABOLIC, PISTACHIO_RINGBREAKER, REAXYS, REAXYS_BIOCATALYSIS database, our tool offers high-accuracy predictions, reflecting the latest in chemical research and data.
Strategy Settings
Precursor scoring | Relevance Heuristic |
---|---|
Min. plausibility | 0.01 |
Model | Template_relevance |
Template Set | Pistachio/Bkms_metabolic/Pistachio_ringbreaker/Reaxys/Reaxys_biocatalysis |
Top-N result to add to graph | 6 |
Feasible Synthetic Routes
Q & A
Q1: What is the role of 1,3-bis(trimethylsilyloxy)propane in the synthesis of (+)-duocarmycin SA?
A: 1,3-Bis(trimethylsilyloxy)propane, also known as 3,7-Dioxa-2,8- disilanonane, 2,2,8,8-tetramethyl-, was investigated as a reagent in the acetalization of hydroxyindoles during the asymmetric total synthesis of (+)-duocarmycin SA. [] The researchers explored different acetalization conditions, and while the specific reaction using 1,3-bis(trimethylsilyloxy)propane wasn't selected for the final synthetic route, it demonstrably yielded the desired alkoxyindole derivative. This highlights the compound's potential utility in protecting group chemistry for complex molecule synthesis.
Q2: Aside from its use in organic synthesis, has 1,3-bis(trimethylsilyloxy)propane been identified in other contexts?
A: Yes, interestingly, 1,3-bis(trimethylsilyloxy)propane was identified as a major component (25.17%) in the bark extract of Juglans regia L., commonly known as the English walnut. [] This finding suggests a potential natural occurrence of the compound and warrants further investigation into its biological significance within the plant.
Q3: Are there alternative reagents to 1,3-bis(trimethylsilyloxy)propane for acetalization reactions in similar synthetic contexts?
A: Yes, the research on (+)-duocarmycin SA synthesis also explored 2-ethyl-2-methyl-1,3-dioxane in combination with boron trifluoride etherate as an alternative acetalization method. [] This method proved successful and was ultimately chosen for the final synthetic route. This highlights the availability of alternative reagents and the importance of evaluating reaction conditions for optimal yield and selectivity in complex molecule synthesis.
Disclaimer and Information on In-Vitro Research Products
Please be aware that all articles and product information presented on BenchChem are intended solely for informational purposes. The products available for purchase on BenchChem are specifically designed for in-vitro studies, which are conducted outside of living organisms. In-vitro studies, derived from the Latin term "in glass," involve experiments performed in controlled laboratory settings using cells or tissues. It is important to note that these products are not categorized as medicines or drugs, and they have not received approval from the FDA for the prevention, treatment, or cure of any medical condition, ailment, or disease. We must emphasize that any form of bodily introduction of these products into humans or animals is strictly prohibited by law. It is essential to adhere to these guidelines to ensure compliance with legal and ethical standards in research and experimentation.