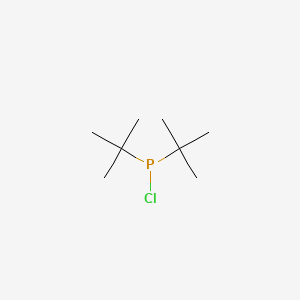
Di-tert-butylchlorophosphine
Overview
Description
Di-tert-butylchlorophosphine is a chemical compound belonging to the class of phosphine ligands. It is known for its flexibility in electronic and steric properties, making it a valuable reagent in various chemical reactions, particularly in cross-coupling reactions. The compound has the molecular formula C8H18ClP and a molecular weight of 180.66 g/mol .
Mechanism of Action
Target of Action
Di-tert-butylchlorophosphine primarily targets the central metal atom in various chemical reactions . It plays a key role in stabilizing and activating the central metal atom, which is crucial for the success of these reactions .
Mode of Action
This compound interacts with its targets by serving as a ligand . It is used for cross-coupling reactions due to the flexibility of its electronic and steric properties . This interaction results in the stabilization and activation of the central metal atom, enabling it to participate in various chemical reactions .
Biochemical Pathways
This compound affects several biochemical pathways. It is used in reactions such as transition metal-catalyzed C-O, C-N, and C-C bond-forming reactions . These reactions are fundamental to many biochemical processes, and the compound’s role in facilitating these reactions underscores its importance in these pathways .
Pharmacokinetics
It is known that the compound is sensitive to air and moisture , which may influence its absorption, distribution, metabolism, and excretion
Result of Action
The molecular and cellular effects of this compound’s action are primarily seen in its role in facilitating chemical reactions. By serving as a ligand and stabilizing the central metal atom, this compound enables the successful execution of various chemical reactions . These reactions can lead to the formation of new compounds, contributing to various biochemical processes .
Action Environment
The action, efficacy, and stability of this compound are influenced by environmental factors. The compound is sensitive to air and moisture , which can affect its stability and reactivity. Therefore, it is typically stored in a dry and well-ventilated place . The temperature and pressure of the environment can also impact the compound’s action, as they can influence the rate and success of the chemical reactions it facilitates .
Biochemical Analysis
Biochemical Properties
Di-tert-butylchlorophosphine plays a key role in stabilizing and activating the central metal atom in biochemical reactions . It is used in transition metal-catalyzed C-O, C-N, and C-C bond-forming reactions
Cellular Effects
It is known that it can cause severe skin burns and eye damage , indicating that it may have significant cytotoxic effects.
Molecular Mechanism
This compound is used as a ligand in the Pd-catalyzed amination reaction with aryl halides and the Pd-catalyzed Suzuki-Miyaura cross-coupling of arylboronic acids with aryl bromides and chlorides
Preparation Methods
Synthetic Routes and Reaction Conditions
Di-tert-butylchlorophosphine can be synthesized through the reaction of tert-butyllithium with phosphorus trichloride. The reaction is typically carried out under an inert atmosphere to prevent the highly reactive intermediates from reacting with moisture or oxygen. The process involves the following steps:
- Preparation of tert-butyllithium by reacting tert-butyl chloride with lithium metal.
- Reaction of tert-butyllithium with phosphorus trichloride to form this compound .
Industrial Production Methods
In industrial settings, the production of this compound follows similar synthetic routes but on a larger scale. The reaction conditions are optimized to ensure high yield and purity of the product. The use of automated systems and controlled environments helps in maintaining the quality and consistency of the compound .
Chemical Reactions Analysis
Types of Reactions
Di-tert-butylchlorophosphine undergoes various types of chemical reactions, including:
Substitution Reactions: It can participate in substitution reactions where the chlorine atom is replaced by other nucleophiles.
Cross-Coupling Reactions: It is widely used in palladium-catalyzed cross-coupling reactions such as Suzuki-Miyaura coupling.
Common Reagents and Conditions
Palladium(II) acetate: Used as a catalyst in cross-coupling reactions.
Arylboronic acids and aryl halides: Common substrates in Suzuki-Miyaura coupling reactions.
Major Products Formed
Aryl-aryl coupled products: Formed in Suzuki-Miyaura coupling reactions.
Substituted phosphines: Formed in substitution reactions.
Scientific Research Applications
Di-tert-butylchlorophosphine has a wide range of applications in scientific research:
Biology: Employed in the synthesis of biologically active molecules.
Medicine: Utilized in the development of pharmaceuticals and drug intermediates.
Industry: Plays a role in the production of polymers and other industrial chemicals.
Comparison with Similar Compounds
Similar Compounds
- Chlorodicyclohexylphosphine
- Chlorodiphenylphosphine
- Tri-tert-butylphosphine
- Chloro(tert-butyl)phenylphosphine
- Di(1-adamantyl)chlorophosphine
- Dicyclohexylphosphine
Uniqueness
Di-tert-butylchlorophosphine is unique due to its high steric bulk and electronic flexibility, which allows it to stabilize a wide range of metal centers and facilitate various catalytic reactions. Its ability to participate in both substitution and cross-coupling reactions makes it a valuable reagent in synthetic chemistry .
Properties
IUPAC Name |
ditert-butyl(chloro)phosphane | |
---|---|---|
Source | PubChem | |
URL | https://pubchem.ncbi.nlm.nih.gov | |
Description | Data deposited in or computed by PubChem | |
InChI |
InChI=1S/C8H18ClP/c1-7(2,3)10(9)8(4,5)6/h1-6H3 | |
Source | PubChem | |
URL | https://pubchem.ncbi.nlm.nih.gov | |
Description | Data deposited in or computed by PubChem | |
InChI Key |
MCRSZLVSRGTMIH-UHFFFAOYSA-N | |
Source | PubChem | |
URL | https://pubchem.ncbi.nlm.nih.gov | |
Description | Data deposited in or computed by PubChem | |
Canonical SMILES |
CC(C)(C)P(C(C)(C)C)Cl | |
Source | PubChem | |
URL | https://pubchem.ncbi.nlm.nih.gov | |
Description | Data deposited in or computed by PubChem | |
Molecular Formula |
C8H18ClP | |
Source | PubChem | |
URL | https://pubchem.ncbi.nlm.nih.gov | |
Description | Data deposited in or computed by PubChem | |
DSSTOX Substance ID |
DTXSID70160089 | |
Record name | Phosphinous chloride, bis(1,1-dimethylethyl)- | |
Source | EPA DSSTox | |
URL | https://comptox.epa.gov/dashboard/DTXSID70160089 | |
Description | DSSTox provides a high quality public chemistry resource for supporting improved predictive toxicology. | |
Molecular Weight |
180.65 g/mol | |
Source | PubChem | |
URL | https://pubchem.ncbi.nlm.nih.gov | |
Description | Data deposited in or computed by PubChem | |
CAS No. |
13716-10-4 | |
Record name | Di-tert-butylchlorophosphine | |
Source | CAS Common Chemistry | |
URL | https://commonchemistry.cas.org/detail?cas_rn=13716-10-4 | |
Description | CAS Common Chemistry is an open community resource for accessing chemical information. Nearly 500,000 chemical substances from CAS REGISTRY cover areas of community interest, including common and frequently regulated chemicals, and those relevant to high school and undergraduate chemistry classes. This chemical information, curated by our expert scientists, is provided in alignment with our mission as a division of the American Chemical Society. | |
Explanation | The data from CAS Common Chemistry is provided under a CC-BY-NC 4.0 license, unless otherwise stated. | |
Record name | Phosphinous chloride, bis(1,1-dimethylethyl)- | |
Source | ChemIDplus | |
URL | https://pubchem.ncbi.nlm.nih.gov/substance/?source=chemidplus&sourceid=0013716104 | |
Description | ChemIDplus is a free, web search system that provides access to the structure and nomenclature authority files used for the identification of chemical substances cited in National Library of Medicine (NLM) databases, including the TOXNET system. | |
Record name | Phosphinous chloride, bis(1,1-dimethylethyl)- | |
Source | EPA DSSTox | |
URL | https://comptox.epa.gov/dashboard/DTXSID70160089 | |
Description | DSSTox provides a high quality public chemistry resource for supporting improved predictive toxicology. | |
Synthesis routes and methods I
Procedure details
Synthesis routes and methods II
Procedure details
Retrosynthesis Analysis
AI-Powered Synthesis Planning: Our tool employs the Template_relevance Pistachio, Template_relevance Bkms_metabolic, Template_relevance Pistachio_ringbreaker, Template_relevance Reaxys, Template_relevance Reaxys_biocatalysis model, leveraging a vast database of chemical reactions to predict feasible synthetic routes.
One-Step Synthesis Focus: Specifically designed for one-step synthesis, it provides concise and direct routes for your target compounds, streamlining the synthesis process.
Accurate Predictions: Utilizing the extensive PISTACHIO, BKMS_METABOLIC, PISTACHIO_RINGBREAKER, REAXYS, REAXYS_BIOCATALYSIS database, our tool offers high-accuracy predictions, reflecting the latest in chemical research and data.
Strategy Settings
Precursor scoring | Relevance Heuristic |
---|---|
Min. plausibility | 0.01 |
Model | Template_relevance |
Template Set | Pistachio/Bkms_metabolic/Pistachio_ringbreaker/Reaxys/Reaxys_biocatalysis |
Top-N result to add to graph | 6 |
Feasible Synthetic Routes
Disclaimer and Information on In-Vitro Research Products
Please be aware that all articles and product information presented on BenchChem are intended solely for informational purposes. The products available for purchase on BenchChem are specifically designed for in-vitro studies, which are conducted outside of living organisms. In-vitro studies, derived from the Latin term "in glass," involve experiments performed in controlled laboratory settings using cells or tissues. It is important to note that these products are not categorized as medicines or drugs, and they have not received approval from the FDA for the prevention, treatment, or cure of any medical condition, ailment, or disease. We must emphasize that any form of bodily introduction of these products into humans or animals is strictly prohibited by law. It is essential to adhere to these guidelines to ensure compliance with legal and ethical standards in research and experimentation.