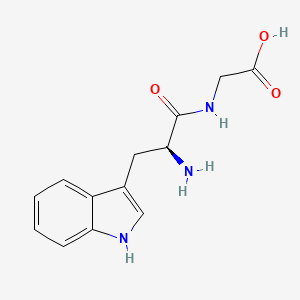
Trp-gly
Overview
Description
Trp-Gly is a dipeptide formed from L-tryptophan and glycine residues. It has a role as a metabolite.
Mechanism of Action
Target of Action
Trp-Gly, also known as H-TRP-GLY-OH, primarily targets the Tryptophan (Trp) metabolic pathways . These pathways play a crucial role in various physiological functions, including inflammation, metabolism, immune responses, and neurological function .
Mode of Action
The compound interacts with its targets through complex metabolic pathways . The interaction results in the production of a variety of bioactive compounds that can regulate various physiological functions .
Biochemical Pathways
This compound affects three main metabolic pathways :
These pathways lead to the degradation of more than 95% of Trp into multiple bioactive compounds .
Pharmacokinetics
It is known that the metabolic stability of similar compounds is limited
Result of Action
The result of this compound’s action is the production of a variety of bioactive compounds via Trp metabolism . These compounds can regulate various physiological functions, including inflammation, metabolism, immune responses, and neurological function .
Action Environment
The action, efficacy, and stability of this compound are influenced by environmental factors. For instance, the gut microbiota and intestine mutually regulate the physiological functions of Trp metabolites to maintain intestinal homeostasis and symbiosis under steady-state conditions and during the immune response to pathogens and xenotoxins .
Biological Activity
Tryptophan-glycine (Trp-Gly) is a dipeptide that has garnered attention for its potential biological activities, particularly in the fields of biochemistry and pharmacology. This article explores the biological activity of this compound, presenting findings from various studies, including data tables and case studies that illustrate its effects and mechanisms of action.
Structure and Properties
This compound consists of two amino acids: tryptophan (Trp), an essential amino acid known for its role in protein synthesis and as a precursor to serotonin, and glycine (Gly), the simplest amino acid that plays a crucial role in various biochemical processes. The molecular structure of this compound allows it to participate in diverse biological interactions.
Antioxidant Properties
Research indicates that this compound exhibits significant antioxidant activity. A study examining the oxidation kinetics of tryptophan residues found that this compound had a higher reactivity index compared to free tryptophan at lower concentrations (5 µM). This suggests that the dipeptide may effectively scavenge free radicals, potentially offering protective effects against oxidative stress .
Table 1: Reactivity Index (R_i) of Tryptophan Residues
Compound | R_i at 5 µM | R_i at 1 mM |
---|---|---|
Free Trp | 1.0 | 1.0 |
This compound | 2.0 | 1.0 |
Gly-Trp-Gly | 2.0 | 1.0 |
Trp-Ala | 1.5 | 1.0 |
Effects on Protein Stability
This compound has been shown to influence protein stability significantly. In experiments with lactose permease (LacY), replacing glycine residues with tryptophan led to alterations in transport activity and thermal stability. Specifically, these replacements resulted in a complete loss of transport activity while enhancing the thermal stability of the protein, suggesting that this compound may stabilize certain protein conformations under stress conditions .
Interaction with Membrane Proteins
The incorporation of Trp into membrane proteins can alter their dynamics and interactions with substrates. In the case of LacY, the introduction of bulky tryptophan residues disrupted critical glycine-glycine interactions, leading to a shift toward an outward-open conformation that affects substrate binding dynamics .
Radical Scavenging Mechanism
The antioxidant mechanism of this compound appears to involve its ability to donate electrons to radical species, thereby neutralizing them. The study on oxidation kinetics demonstrated that dipeptides, including this compound, exhibited enhanced reactivity compared to their free amino acid counterparts at lower concentrations, indicating a potential mechanism for their antioxidant properties .
Study on Antioxidant Activity
A study conducted by researchers assessed the antioxidant capabilities of various peptides, including this compound. The findings indicated that at low concentrations, this compound showed twice the reactivity compared to free tryptophan, highlighting its potential as an effective antioxidant agent .
Protein Stability Analysis
In another research study focusing on LacY mutants with Trp substitutions, it was observed that these modifications significantly impacted both sugar binding rates and overall protein stability. The results suggested that such dipeptide modifications could be utilized to engineer proteins with desired stability characteristics for therapeutic applications .
Scientific Research Applications
Biochemical Research
1.1 Oxidation Mechanisms
Trp-Gly plays a significant role in studies investigating the oxidation of tryptophan residues. Research has shown that Trp residues can undergo oxidation mediated by peroxyl radicals, leading to complex reactions involving this compound and other dipeptides. At low concentrations, this compound exhibits enhanced reactivity compared to free tryptophan, indicating its potential as a model compound for understanding oxidative processes in proteins and peptides .
1.2 Fluorescence Studies
Fluorescence spectroscopy has been employed to study the structural dynamics of this compound and its implications in protein interactions. The dipeptide's rotamer structure has been correlated with fluorescence emission shifts, providing insights into its conformational behavior in various environments . This understanding is crucial for elucidating the role of tryptophan in protein folding and stability.
Agricultural Applications
2.1 Plant Growth Enhancement
Recent studies have highlighted the potential of this compound in promoting plant growth and enhancing crop yield. Tryptophan, a precursor to indole-3-acetic acid (IAA), is vital for plant hormone synthesis. Research indicates that foliar applications of amino acids, including Trp and glycine, can significantly improve chlorophyll content and overall plant health in crops like celery . The combination of Trp and glycine has been shown to enhance vegetative growth characteristics and increase nutrient content in plants.
Treatment Concentration (mg/L) | Plant Height Increase (%) | Yield Increase (%) |
---|---|---|
75 mg/L (Trp) | 16.9 | 58.4 |
75 mg/L (Trp + Gly) | Significant improvement | Not specified |
Medicinal Chemistry
3.1 Antioxidant Properties
This compound has been investigated for its antioxidant properties, contributing to the development of antioxidant peptides that can alleviate oxidative stress-related diseases. Antioxidant peptides derived from various sources demonstrate significant scavenging activity against free radicals, which can be beneficial in therapeutic applications . The specific structural features of this compound may enhance its efficacy as an antioxidant agent.
3.2 Photochemical Reactions
The photochemical behavior of this compound has been explored through studies on light-induced transformations in immunoglobulins. Exposure to specific wavelengths leads to the fragmentation of tryptophan into glycine and glycine hydroperoxide, suggesting potential applications in photodynamic therapy and targeted drug delivery systems . This reaction pathway underscores the importance of this compound in developing innovative therapeutic strategies.
Chemical Reactions Analysis
Formation of Trp-Gly
- Peptide bond formation A peptide bond is created through a dehydration-condensation reaction, where the amine group of one amino acid reacts with the carboxylic acid of another, resulting in the formation of a CO-NH amide group and the release of water. When tryptophan combines with glycine, it forms either this compound or Gly-Trp .
- Enzymatic Synthesis A study of peptide prebiotic synthesis indicated that Gly-Gly, this compound, Gly-Trp, Trp-Trp, and Trp-Trp-Trp were the main reaction products for the experimental mixture glycine + tryptophan .
- Cyclic peptide synthesis Cyclo(-Gly-Trp) is a building block in the synthesis of cyclic peptides, important in drug development due to their stability and bioactivity .
Oxidation
- Free Radical Scavenging Tryptophan and tyrosine exhibit antioxidant activity because of the capacity of their phenolic and indolic groups to serve as hydrogen donors . Studies using ABTS, DPPH, and ORAC assays revealed the free radical scavenging activities of tripeptide libraries. Tryptophan and tyrosine groups had higher activity than histidine groups .
- AAPH Radical scavenging Tryptophan residues can scavenge AAPH radicals efficiently .
- Oxidation by AAPH-derived free radicals The kinetics of oxidation of free, peptide, and protein tryptophan residues by AAPH-derived free radicals has been investigated. At low tryptophan concentrations (5 μM), di- and tripeptides had R<sub>i</sub> values twice those of free tryptophan, whereas at high concentrations (1 mM), all compounds showed similar R<sub>i</sub> values .
- Role of Alkoxyl Radicals At low tryptophan concentrations, alkoxyl radicals are responsible for tryptophan oxidation, while at high tryptophan concentrations, a mixture of peroxyl and alkoxyl radicals are involved .
Photochemical Reactions
- Light-induced Fragmentation Exposure of IgG1 to light results in the fragmentation of tryptophan, leading to the formation of glycine and/or glycine hydroperoxide .
- AlphaC- betaC side chain fragmentation During the exposure of IgG1 to light with λ = 254 nm, fragmentation of Trp[191, LC] resulted in the formation of Gly and Gly hydroperoxide .
Interactions with other molecules
-
Cucurbituril Hosts The synthetic receptors cucurbituril (Q) can recognize peptides and proteins. Cucurbit uril (Q8) can bind to peptides containing tryptophan residues .
Host Peptide or protein K a (M-1) ΔH (kcal mol-1) −TΔS (kcal mol-1) Q8·MV a H-Trp-Gly-Gly-OH 1.3 × 10<sup>5</sup> −14.8 7.8 Q8·MV a H-Gly-Trp-Gly-OH 2.1 × 10<sup>4</sup> −11.4 5.5 Q8·MV a H-Gly-Gly-Trp-OH 3.1 × 10<sup>3</sup> −8.8 4.0 Q8·MV a H-Gly-Gly-Trp-Gly-Gly-OH 2.5 × 10<sup>4</sup> −12.1 6.1 Q8·MBBI f H-Trp-Gly-Gly-OH 1.2 × 10<sup>5</sup> −15.6 8.6 Q8·MBBI f H-Gly-Trp-Gly-OH 1.7 × 10<sup>4</sup> −16.5 10.7 Q8·MBBI f H-Gly-Gly-Trp-OH 4.1 × 10<sup>3</sup> −13.0 8.0 a 10 mM sodium phosphate, pH 7.0, 300 K. f 10 mM sodium phosphate, pH 7.0, 300 K. - DNA Interactions Tyrosyl, histidyl, and tryptophanyl peptides interact with DNA .
Other reactions
- Electrochemical Bioconjugation Tryptophan can be used for electrochemical bioconjugation reactions to generate artificial peptides and proteins .
- Nitration Tryptophan derivatives can undergo enzymatic nitration by oxidation of nitrite using lactoperoxidase and horseradish peroxidase .
- Conversion to Glycine The light exposure of selected peptides and monoclonal antibodies leads to the conversion of tryptophan to glycine .
- Self-association this compound-Trp fragments self-associate in water .
- Hydrolysis this compound can be broken down into its constituent amino acids, tryptophan and glycine, through hydrolysis .
Q & A
Q. How can researchers determine the structural stability of Trp-Gly in aqueous environments, and what experimental methods are most reliable?
Basic Research Focus
To assess this compound’s conformational stability, employ spectroscopic techniques such as circular dichroism (CD) or nuclear magnetic resonance (NMR) to analyze peptide folding in varying pH and ionic conditions. Computational methods like molecular dynamics (MD) simulations can predict solvent interactions and stability . For validation, cross-reference results with empirical data from infrared (IR) spectroscopy , which identifies hydrogen-bonding patterns critical to structural integrity .
Q. What methodological steps are required to resolve contradictions in reported PEPT1 transporter affinity for this compound across different experimental models?
Advanced Research Focus
Contradictions often arise from variations in experimental models (e.g., in vitro cell lines vs. in vivo systems). To address this:
Standardize assay conditions : Control temperature (e.g., 37°C for physiological relevance vs. 4°C for passive diffusion studies) and buffer composition .
Validate transporter specificity : Use competitive inhibitors (e.g., Gly-Sar) to confirm PEPT1-mediated uptake .
Leverage knockout models : Compare wild-type and PEPT1-deficient systems to isolate transporter contributions .
Apply statistical rigor : Use ANOVA to analyze variance between replicates and conditions .
Table 1 : Intracellular accumulation of this compound under varying conditions
Condition | 37°C Accumulation (%) | 4°C Accumulation (%) |
---|---|---|
This compound | 85 ± 3.2 | 22 ± 1.5 |
Gly-Sar | 65 ± 2.8 | 18 ± 1.1 |
Valacyclovir | 60 ± 2.5 | 15 ± 0.9 |
Q. How can computational methods like RI-DFT-D be optimized to predict this compound’s vibrational spectra with high accuracy?
Advanced Research Focus
Benchmark against high-level theories : Compare RI-DFT-D (TPSS/TZVP) results with CCSD(T) -derived data to validate accuracy .
Incorporate dispersion corrections : Use damped empirical dispersion terms to account for weak intermolecular interactions in peptide chains .
Calibrate with experimental IR spectra : Assign calculated vibrational frequencies to observed peaks, ensuring alignment within ±10 cm⁻¹ .
Optimize computational efficiency : Balance accuracy with CPU time by testing basis sets (e.g., TZVP vs. cc-pVTZ) .
Q. What are the best practices for designing in vitro studies to evaluate this compound’s membrane permeability?
Basic Research Focus
Select appropriate models : Use Caco-2 cells or artificial lipid bilayers for passive diffusion assays .
Control confounding factors : Maintain consistent pH (6.5–7.4) and exclude serum proteins that may bind peptides .
Quantify uptake kinetics : Apply LC-MS/MS for precise measurement of intracellular this compound concentrations .
Adhere to NIH guidelines : Document cell viability, passage numbers, and assay validation steps to ensure reproducibility .
Q. How should researchers address discrepancies between computational predictions and empirical data on this compound’s bioactive conformers?
Advanced Research Focus
Reconcile sampling limitations : Extend MD simulation durations (>100 ns) to capture rare conformational states .
Integrate multi-method data : Combine NMR-derived NOE restraints with MD trajectories to refine conformational ensembles .
Evaluate force field accuracy : Test AMBER vs. CHARMM parameters for peptide backbone flexibility .
Publish negative results : Document non-aligned predictions to improve community-wide model calibration .
Q. What statistical approaches are recommended for analyzing dose-response relationships in this compound’s cellular uptake studies?
Basic Research Focus
Non-linear regression : Fit data to Michaelis-Menten models to estimate and .
Outlier detection : Use Grubbs’ test to exclude anomalous data points from transport assays .
Meta-analysis : Pool data from multiple studies to identify trends in transporter affinity .
Q. How can researchers ensure ethical and reproducible preclinical studies on this compound’s pharmacokinetics?
Basic Research Focus
Follow ARRIVE guidelines : Report animal strain, sample size, and randomization protocols .
Validate analytical methods : Include internal standards (e.g., stable isotope-labeled this compound) in LC-MS workflows .
Archive raw data : Share chromatograms and kinetic plots in public repositories (e.g., Zenodo) .
Q. Key Recommendations for Methodological Rigor
- Cross-validate findings : Use orthogonal methods (e.g., computational + experimental) to confirm results .
- Prioritize transparency : Document all experimental parameters and statistical thresholds in supplementary materials .
- Engage peer review early : Pre-publish protocols on platforms like bioRxiv to solicit feedback on study design .
Properties
IUPAC Name |
2-[[2-amino-3-(1H-indol-3-yl)propanoyl]amino]acetic acid | |
---|---|---|
Details | Computed by Lexichem TK 2.7.0 (PubChem release 2021.05.07) | |
Source | PubChem | |
URL | https://pubchem.ncbi.nlm.nih.gov | |
Description | Data deposited in or computed by PubChem | |
InChI |
InChI=1S/C13H15N3O3/c14-10(13(19)16-7-12(17)18)5-8-6-15-11-4-2-1-3-9(8)11/h1-4,6,10,15H,5,7,14H2,(H,16,19)(H,17,18) | |
Details | Computed by InChI 1.0.6 (PubChem release 2021.05.07) | |
Source | PubChem | |
URL | https://pubchem.ncbi.nlm.nih.gov | |
Description | Data deposited in or computed by PubChem | |
InChI Key |
UYKREHOKELZSPB-UHFFFAOYSA-N | |
Details | Computed by InChI 1.0.6 (PubChem release 2021.05.07) | |
Source | PubChem | |
URL | https://pubchem.ncbi.nlm.nih.gov | |
Description | Data deposited in or computed by PubChem | |
Canonical SMILES |
C1=CC=C2C(=C1)C(=CN2)CC(C(=O)NCC(=O)O)N | |
Details | Computed by OEChem 2.3.0 (PubChem release 2021.05.07) | |
Source | PubChem | |
URL | https://pubchem.ncbi.nlm.nih.gov | |
Description | Data deposited in or computed by PubChem | |
Molecular Formula |
C13H15N3O3 | |
Details | Computed by PubChem 2.1 (PubChem release 2021.05.07) | |
Source | PubChem | |
URL | https://pubchem.ncbi.nlm.nih.gov | |
Description | Data deposited in or computed by PubChem | |
Molecular Weight |
261.28 g/mol | |
Details | Computed by PubChem 2.1 (PubChem release 2021.05.07) | |
Source | PubChem | |
URL | https://pubchem.ncbi.nlm.nih.gov | |
Description | Data deposited in or computed by PubChem | |
Physical Description |
Solid | |
Record name | Tryptophyl-Glycine | |
Source | Human Metabolome Database (HMDB) | |
URL | http://www.hmdb.ca/metabolites/HMDB0029083 | |
Description | The Human Metabolome Database (HMDB) is a freely available electronic database containing detailed information about small molecule metabolites found in the human body. | |
Explanation | HMDB is offered to the public as a freely available resource. Use and re-distribution of the data, in whole or in part, for commercial purposes requires explicit permission of the authors and explicit acknowledgment of the source material (HMDB) and the original publication (see the HMDB citing page). We ask that users who download significant portions of the database cite the HMDB paper in any resulting publications. | |
CAS No. |
7360-09-0 | |
Record name | Glycine, N-L-tryptophyl- | |
Source | DTP/NCI | |
URL | https://dtp.cancer.gov/dtpstandard/servlet/dwindex?searchtype=NSC&outputformat=html&searchlist=97945 | |
Description | The NCI Development Therapeutics Program (DTP) provides services and resources to the academic and private-sector research communities worldwide to facilitate the discovery and development of new cancer therapeutic agents. | |
Explanation | Unless otherwise indicated, all text within NCI products is free of copyright and may be reused without our permission. Credit the National Cancer Institute as the source. | |
Retrosynthesis Analysis
AI-Powered Synthesis Planning: Our tool employs the Template_relevance Pistachio, Template_relevance Bkms_metabolic, Template_relevance Pistachio_ringbreaker, Template_relevance Reaxys, Template_relevance Reaxys_biocatalysis model, leveraging a vast database of chemical reactions to predict feasible synthetic routes.
One-Step Synthesis Focus: Specifically designed for one-step synthesis, it provides concise and direct routes for your target compounds, streamlining the synthesis process.
Accurate Predictions: Utilizing the extensive PISTACHIO, BKMS_METABOLIC, PISTACHIO_RINGBREAKER, REAXYS, REAXYS_BIOCATALYSIS database, our tool offers high-accuracy predictions, reflecting the latest in chemical research and data.
Strategy Settings
Precursor scoring | Relevance Heuristic |
---|---|
Min. plausibility | 0.01 |
Model | Template_relevance |
Template Set | Pistachio/Bkms_metabolic/Pistachio_ringbreaker/Reaxys/Reaxys_biocatalysis |
Top-N result to add to graph | 6 |
Feasible Synthetic Routes
Disclaimer and Information on In-Vitro Research Products
Please be aware that all articles and product information presented on BenchChem are intended solely for informational purposes. The products available for purchase on BenchChem are specifically designed for in-vitro studies, which are conducted outside of living organisms. In-vitro studies, derived from the Latin term "in glass," involve experiments performed in controlled laboratory settings using cells or tissues. It is important to note that these products are not categorized as medicines or drugs, and they have not received approval from the FDA for the prevention, treatment, or cure of any medical condition, ailment, or disease. We must emphasize that any form of bodily introduction of these products into humans or animals is strictly prohibited by law. It is essential to adhere to these guidelines to ensure compliance with legal and ethical standards in research and experimentation.