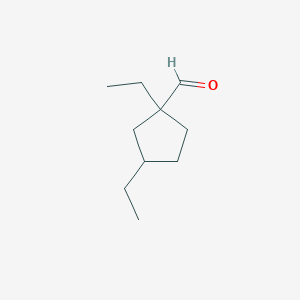
1,3-Diethylcyclopentane-1-carbaldehyde
- Click on QUICK INQUIRY to receive a quote from our team of experts.
- With the quality product at a COMPETITIVE price, you can focus more on your research.
Overview
Description
1,3-Diethylcyclopentane-1-carbaldehyde is an organic compound with the molecular formula C10H18O. It is a cyclopentane derivative where two ethyl groups are attached to the first and third carbon atoms of the cyclopentane ring, and an aldehyde functional group is attached to the first carbon atom. This compound is of interest in various fields of chemistry due to its unique structure and reactivity.
Preparation Methods
Synthetic Routes and Reaction Conditions
1,3-Diethylcyclopentane-1-carbaldehyde can be synthesized through several methods. One common approach involves the alkylation of cyclopentanone followed by a formylation reaction. The general steps are as follows:
Alkylation of Cyclopentanone: Cyclopentanone is reacted with ethyl bromide in the presence of a strong base such as sodium hydride (NaH) to introduce the ethyl groups at the 1 and 3 positions of the cyclopentane ring.
Formylation: The resulting 1,3-diethylcyclopentane is then subjected to formylation using reagents like dichloromethyl methyl ether (Cl2CHOCH3) and a Lewis acid catalyst such as titanium tetrachloride (TiCl4) to introduce the aldehyde group at the 1 position.
Industrial Production Methods
In an industrial setting, the production of this compound may involve continuous flow processes to ensure high yield and purity. The use of automated reactors and precise control of reaction conditions (temperature, pressure, and reagent concentration) can optimize the synthesis.
Chemical Reactions Analysis
Types of Reactions
1,3-Diethylcyclopentane-1-carbaldehyde undergoes various chemical reactions, including:
Oxidation: The aldehyde group can be oxidized to a carboxylic acid using oxidizing agents such as potassium permanganate (KMnO4) or chromium trioxide (CrO3).
Reduction: The aldehyde group can be reduced to a primary alcohol using reducing agents like sodium borohydride (NaBH4) or lithium aluminum hydride (LiAlH4).
Substitution: The ethyl groups can undergo substitution reactions, such as halogenation, where a halogen atom replaces one of the hydrogen atoms on the ethyl group.
Common Reagents and Conditions
Oxidation: KMnO4 in an aqueous medium or CrO3 in acetic acid.
Reduction: NaBH4 in methanol or LiAlH4 in ether.
Substitution: Halogenation using bromine (Br2) or chlorine (Cl2) in the presence of a radical initiator.
Major Products
Oxidation: 1,3-Diethylcyclopentane-1-carboxylic acid.
Reduction: 1,3-Diethylcyclopentane-1-methanol.
Substitution: 1,3-Diethylcyclopentane-1-bromoethane or 1,3-Diethylcyclopentane-1-chloroethane.
Scientific Research Applications
1,3-Diethylcyclopentane-1-carbaldehyde has several applications in scientific research:
Chemistry: It is used as a building block in organic synthesis for the preparation of more complex molecules.
Biology: It can be used in the study of enzyme-catalyzed reactions involving aldehydes.
Industry: Used in the synthesis of specialty chemicals and intermediates for various industrial processes.
Mechanism of Action
The mechanism of action of 1,3-diethylcyclopentane-1-carbaldehyde involves its reactivity as an aldehyde. The aldehyde group can participate in nucleophilic addition reactions, where nucleophiles attack the carbonyl carbon, leading to the formation of various adducts. The molecular targets and pathways involved depend on the specific reactions and applications in which the compound is used.
Comparison with Similar Compounds
Similar Compounds
1,3-Diethylcyclopentane: Lacks the aldehyde group, making it less reactive in nucleophilic addition reactions.
Cyclopentanone: Contains a ketone group instead of an aldehyde, leading to different reactivity and applications.
1,3-Dimethylcyclopentane-1-carbaldehyde: Similar structure but with methyl groups instead of ethyl groups, affecting its physical and chemical properties.
Uniqueness
1,3-Diethylcyclopentane-1-carbaldehyde is unique due to the presence of both ethyl groups and an aldehyde functional group on the cyclopentane ring. This combination of substituents imparts distinct reactivity and makes it a valuable compound in various chemical transformations and applications.
Biological Activity
1,3-Diethylcyclopentane-1-carbaldehyde (DECPC) is a cyclopentane derivative that has garnered attention in various fields of research due to its potential biological activities. This compound is characterized by its unique structure, which influences its reactivity and interactions with biological systems. This article explores the biological activity of DECPC, summarizing key findings from diverse sources, including case studies and experimental data.
Chemical Structure and Properties
DECPC has the following molecular formula:
- Molecular Formula : C₉H₁₄O
- CAS Number : Not specifically listed but can be identified through chemical databases.
The compound's structure features a cyclopentane ring with two ethyl groups and an aldehyde functional group, which may contribute to its biological properties.
Biological Activity Overview
Research on DECPC indicates various biological activities, including antimicrobial, antifungal, and potential neuroprotective effects. The aldehyde group is particularly relevant as it can participate in nucleophilic addition reactions, potentially interacting with biomolecules such as proteins and nucleic acids.
Antimicrobial Activity
Several studies have highlighted the antimicrobial properties of DECPC. For instance, a study examining the volatile emissions from decomposing organic matter indicated that compounds like DECPC play a significant role in attracting specific insect species that aid in decomposition processes . This suggests a potential ecological role in microbial interactions.
Antifungal Activity
In laboratory settings, DECPC has demonstrated antifungal properties against various fungal strains. The mechanism is believed to involve the disruption of fungal cell membranes or interference with metabolic pathways. Such findings are critical for developing new antifungal agents.
1. Insect Attraction Studies
A notable case study involved the analysis of volatile compounds emitted from decomposing cadavers. DECPC was found to constitute approximately 50% of the total volatile emissions over a 36-day period . This high concentration indicates its significant role in chemical communication within ecosystems, particularly in attracting carrion beetles that contribute to decomposition.
Compound | Percentage of Total Volatiles |
---|---|
This compound | ~50% |
Other Compounds | Various (including carboxylic acids) |
2. Antifungal Efficacy
In a controlled laboratory experiment, DECPC was tested against common fungal pathogens. Results indicated that DECPC inhibited fungal growth at concentrations as low as 100 µg/mL. The study concluded that further exploration into its mechanism could lead to novel antifungal therapies.
The biological activity of DECPC may be attributed to its ability to form reactive intermediates upon interaction with biological nucleophiles. The aldehyde group can undergo reactions such as:
- Nucleophilic Addition : Reacting with amines or thiols to form stable adducts.
- Oxidation : Potentially transforming into carboxylic acids that may have different biological activities.
These mechanisms underscore the compound's versatility and potential applications in drug development and environmental science.
Properties
Molecular Formula |
C10H18O |
---|---|
Molecular Weight |
154.25 g/mol |
IUPAC Name |
1,3-diethylcyclopentane-1-carbaldehyde |
InChI |
InChI=1S/C10H18O/c1-3-9-5-6-10(4-2,7-9)8-11/h8-9H,3-7H2,1-2H3 |
InChI Key |
YDJXBEHBZKOHST-UHFFFAOYSA-N |
Canonical SMILES |
CCC1CCC(C1)(CC)C=O |
Origin of Product |
United States |
Disclaimer and Information on In-Vitro Research Products
Please be aware that all articles and product information presented on BenchChem are intended solely for informational purposes. The products available for purchase on BenchChem are specifically designed for in-vitro studies, which are conducted outside of living organisms. In-vitro studies, derived from the Latin term "in glass," involve experiments performed in controlled laboratory settings using cells or tissues. It is important to note that these products are not categorized as medicines or drugs, and they have not received approval from the FDA for the prevention, treatment, or cure of any medical condition, ailment, or disease. We must emphasize that any form of bodily introduction of these products into humans or animals is strictly prohibited by law. It is essential to adhere to these guidelines to ensure compliance with legal and ethical standards in research and experimentation.