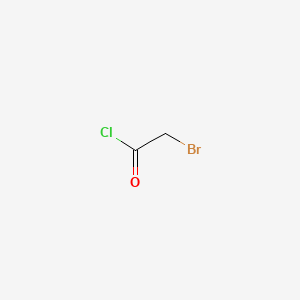
Bromoacetyl chloride
Overview
Description
Bromoacetyl chloride is an organic compound with the molecular formula C₂H₂BrClO. It is a colorless to pale yellow liquid with a pungent odor. This compound is primarily used as an intermediate in organic synthesis, particularly in the preparation of various pharmaceuticals and agrochemicals .
Preparation Methods
Synthetic Routes and Reaction Conditions: Bromoacetyl chloride can be synthesized through the bromination of acetyl chloride. The reaction typically involves the use of bromine (Br₂) in the presence of a catalyst such as iron (Fe) or aluminum bromide (AlBr₃). The reaction is carried out under controlled conditions to ensure the selective formation of this compound .
Industrial Production Methods: In an industrial setting, this compound is produced by the direct bromination of acetyl chloride using bromine. The process is optimized to achieve high yields and purity of the product. The reaction is typically conducted in a continuous flow reactor to maintain consistent reaction conditions and to facilitate large-scale production .
Chemical Reactions Analysis
Types of Reactions: Bromoacetyl chloride undergoes various chemical reactions, including:
Nucleophilic Substitution: It reacts with nucleophiles such as amines, alcohols, and thiols to form corresponding substituted products.
Hydrolysis: In the presence of water, this compound hydrolyzes to form bromoacetic acid and hydrochloric acid.
Reduction: It can be reduced to bromoacetaldehyde using reducing agents like lithium aluminum hydride (LiAlH₄).
Common Reagents and Conditions:
Nucleophilic Substitution: Reagents such as amines, alcohols, and thiols are commonly used. The reactions are typically carried out in an inert solvent like dichloromethane or tetrahydrofuran (THF) at room temperature.
Hydrolysis: Water or aqueous solutions are used under mild conditions.
Reduction: Lithium aluminum hydride (LiAlH₄) is used in anhydrous ether or THF under controlled conditions.
Major Products Formed:
Nucleophilic Substitution: Substituted amides, esters, and thioesters.
Hydrolysis: Bromoacetic acid and hydrochloric acid.
Reduction: Bromoacetaldehyde.
Scientific Research Applications
Bromoacetyl chloride has several applications in scientific research:
Chemistry: It is used as a reagent in the synthesis of various organic compounds, including pharmaceuticals and agrochemicals.
Biology: It is employed in the modification of biomolecules, such as proteins and nucleic acids, to study their structure and function.
Medicine: this compound is used in the synthesis of active pharmaceutical ingredients (APIs) and intermediates for drug development.
Industry: It is utilized in the production of specialty chemicals and materials, including polymers and resins.
Mechanism of Action
The mechanism of action of bromoacetyl chloride involves its reactivity as an acylating agent. It reacts with nucleophiles, such as amines and alcohols, to form acylated products. The molecular targets include functional groups containing nucleophilic atoms like nitrogen, oxygen, and sulfur. The pathways involved in these reactions typically follow a nucleophilic substitution mechanism, where the nucleophile attacks the carbonyl carbon of this compound, leading to the displacement of the bromine atom .
Comparison with Similar Compounds
Chloroacetyl chloride (C₂H₂Cl₂O): Similar in structure but contains a chlorine atom instead of a bromine atom.
Bromoacetyl bromide (C₂H₂Br₂O): Contains two bromine atoms instead of one.
3-Bromopropionyl chloride (C₃H₄BrClO): Contains an additional carbon atom in the chain.
Comparison:
Reactivity: Bromoacetyl chloride is more reactive than chloroacetyl chloride due to the presence of the bromine atom, which is a better leaving group.
Selectivity: this compound offers higher selectivity in nucleophilic substitution reactions compared to bromoacetyl bromide, which can lead to multiple substitution products.
Applications: While all these compounds are used in organic synthesis, this compound is preferred for specific applications requiring high reactivity and selectivity
Properties
IUPAC Name |
2-bromoacetyl chloride | |
---|---|---|
Source | PubChem | |
URL | https://pubchem.ncbi.nlm.nih.gov | |
Description | Data deposited in or computed by PubChem | |
InChI |
InChI=1S/C2H2BrClO/c3-1-2(4)5/h1H2 | |
Source | PubChem | |
URL | https://pubchem.ncbi.nlm.nih.gov | |
Description | Data deposited in or computed by PubChem | |
InChI Key |
SYZRZLUNWVNNNV-UHFFFAOYSA-N | |
Source | PubChem | |
URL | https://pubchem.ncbi.nlm.nih.gov | |
Description | Data deposited in or computed by PubChem | |
Canonical SMILES |
C(C(=O)Cl)Br | |
Source | PubChem | |
URL | https://pubchem.ncbi.nlm.nih.gov | |
Description | Data deposited in or computed by PubChem | |
Molecular Formula |
C2H2BrClO | |
Source | PubChem | |
URL | https://pubchem.ncbi.nlm.nih.gov | |
Description | Data deposited in or computed by PubChem | |
DSSTOX Substance ID |
DTXSID80176636 | |
Record name | Bromoacetyl chloride | |
Source | EPA DSSTox | |
URL | https://comptox.epa.gov/dashboard/DTXSID80176636 | |
Description | DSSTox provides a high quality public chemistry resource for supporting improved predictive toxicology. | |
Molecular Weight |
157.39 g/mol | |
Source | PubChem | |
URL | https://pubchem.ncbi.nlm.nih.gov | |
Description | Data deposited in or computed by PubChem | |
CAS No. |
22118-09-8 | |
Record name | Bromoacetyl chloride | |
Source | CAS Common Chemistry | |
URL | https://commonchemistry.cas.org/detail?cas_rn=22118-09-8 | |
Description | CAS Common Chemistry is an open community resource for accessing chemical information. Nearly 500,000 chemical substances from CAS REGISTRY cover areas of community interest, including common and frequently regulated chemicals, and those relevant to high school and undergraduate chemistry classes. This chemical information, curated by our expert scientists, is provided in alignment with our mission as a division of the American Chemical Society. | |
Explanation | The data from CAS Common Chemistry is provided under a CC-BY-NC 4.0 license, unless otherwise stated. | |
Record name | Bromoacetyl chloride | |
Source | ChemIDplus | |
URL | https://pubchem.ncbi.nlm.nih.gov/substance/?source=chemidplus&sourceid=0022118098 | |
Description | ChemIDplus is a free, web search system that provides access to the structure and nomenclature authority files used for the identification of chemical substances cited in National Library of Medicine (NLM) databases, including the TOXNET system. | |
Record name | Bromoacetyl chloride | |
Source | DTP/NCI | |
URL | https://dtp.cancer.gov/dtpstandard/servlet/dwindex?searchtype=NSC&outputformat=html&searchlist=97376 | |
Description | The NCI Development Therapeutics Program (DTP) provides services and resources to the academic and private-sector research communities worldwide to facilitate the discovery and development of new cancer therapeutic agents. | |
Explanation | Unless otherwise indicated, all text within NCI products is free of copyright and may be reused without our permission. Credit the National Cancer Institute as the source. | |
Record name | Bromoacetyl chloride | |
Source | EPA DSSTox | |
URL | https://comptox.epa.gov/dashboard/DTXSID80176636 | |
Description | DSSTox provides a high quality public chemistry resource for supporting improved predictive toxicology. | |
Record name | Bromoacetyl chloride | |
Source | European Chemicals Agency (ECHA) | |
URL | https://echa.europa.eu/substance-information/-/substanceinfo/100.040.704 | |
Description | The European Chemicals Agency (ECHA) is an agency of the European Union which is the driving force among regulatory authorities in implementing the EU's groundbreaking chemicals legislation for the benefit of human health and the environment as well as for innovation and competitiveness. | |
Explanation | Use of the information, documents and data from the ECHA website is subject to the terms and conditions of this Legal Notice, and subject to other binding limitations provided for under applicable law, the information, documents and data made available on the ECHA website may be reproduced, distributed and/or used, totally or in part, for non-commercial purposes provided that ECHA is acknowledged as the source: "Source: European Chemicals Agency, http://echa.europa.eu/". Such acknowledgement must be included in each copy of the material. ECHA permits and encourages organisations and individuals to create links to the ECHA website under the following cumulative conditions: Links can only be made to webpages that provide a link to the Legal Notice page. | |
Retrosynthesis Analysis
AI-Powered Synthesis Planning: Our tool employs the Template_relevance Pistachio, Template_relevance Bkms_metabolic, Template_relevance Pistachio_ringbreaker, Template_relevance Reaxys, Template_relevance Reaxys_biocatalysis model, leveraging a vast database of chemical reactions to predict feasible synthetic routes.
One-Step Synthesis Focus: Specifically designed for one-step synthesis, it provides concise and direct routes for your target compounds, streamlining the synthesis process.
Accurate Predictions: Utilizing the extensive PISTACHIO, BKMS_METABOLIC, PISTACHIO_RINGBREAKER, REAXYS, REAXYS_BIOCATALYSIS database, our tool offers high-accuracy predictions, reflecting the latest in chemical research and data.
Strategy Settings
Precursor scoring | Relevance Heuristic |
---|---|
Min. plausibility | 0.01 |
Model | Template_relevance |
Template Set | Pistachio/Bkms_metabolic/Pistachio_ringbreaker/Reaxys/Reaxys_biocatalysis |
Top-N result to add to graph | 6 |
Feasible Synthetic Routes
Q1: What is the molecular formula and weight of bromoacetyl chloride?
A1: this compound has the molecular formula C2H2BrClO and a molecular weight of 173.40 g/mol. []
Q2: What spectroscopic data is available for this compound?
A2: Numerous spectroscopic studies have been conducted on this compound. Researchers have utilized techniques such as Raman spectroscopy, infrared spectroscopy, and electron diffraction to study its structure and properties. [, , , ]
Q3: Does this compound exhibit conformational isomerism?
A3: Yes, this compound exists as two rotational isomers in the liquid and gaseous states: a more stable trans form and a less stable gauche form. The energy difference between these isomers has been determined through temperature-dependent infrared spectroscopy. [, , ]
Q4: How does this compound behave upon photoexcitation in the ultraviolet region?
A4: Upon excitation at 248 nm, this compound undergoes photodissociation primarily through C–Cl and C–Br bond fission. Interestingly, despite the C–Br bond being weaker, C–Cl bond fission is often favored. [, , , , , ]
Q5: What role do nonadiabatic effects play in the photodissociation of this compound?
A5: Nonadiabatic effects, particularly recrossing at the avoided crossing between the S1 and S2 excited states, significantly influence the branching ratio between C–Cl and C–Br bond fission. Theoretical studies suggest that while nonadiabaticity initially hinders C–Br fission, the dissociation eventually proceeds along a diabatic pathway to excited-state products. [, , , , ]
Q6: How does the distance between the carbonyl group and the C–Br bond affect the photodissociation dynamics?
A6: Studies comparing this compound with bromopropionyl chloride (which has an additional CH2 spacer) reveal that increasing the distance between the carbonyl group and the C–Br bond reduces the energy difference between adiabatic potential surfaces, further suppressing C–Br bond fission. []
Q7: What are some applications of this compound in organic synthesis?
A7: this compound serves as a versatile reagent in organic synthesis. It is employed in reactions such as Friedel-Crafts acylation, nucleophilic substitution, and the synthesis of heterocyclic compounds. Specific examples include the synthesis of tert-butyl 2-bromoacetate, levalbuerol, and tellurium-containing heterocycles. [, , , , ]
Q8: Can this compound be used to modify polymers?
A8: Yes, this compound is utilized to modify polymers such as chitosan. The resulting haloacetylated chitosan derivatives can be further quaternized to impart antimicrobial properties. []
Q9: How have computational methods been employed to study this compound?
A9: Computational chemistry techniques, including ab initio calculations, density functional theory, and molecular dynamics simulations, have provided valuable insights into the potential energy surfaces, reaction pathways, and nonadiabatic dynamics of this compound photodissociation. [, , , ]
Q10: Has this compound been used in the context of quantum computing?
A10: Theoretical studies have explored the potential of using this compound for vibrational quantum computing. Its four bright infrared-active modes make it a potential candidate for encoding qubits. []
Q11: What safety precautions should be taken when handling this compound?
A11: this compound is a reactive chemical and should be handled with caution. Appropriate personal protective equipment, including gloves and eye protection, should be worn. []
Disclaimer and Information on In-Vitro Research Products
Please be aware that all articles and product information presented on BenchChem are intended solely for informational purposes. The products available for purchase on BenchChem are specifically designed for in-vitro studies, which are conducted outside of living organisms. In-vitro studies, derived from the Latin term "in glass," involve experiments performed in controlled laboratory settings using cells or tissues. It is important to note that these products are not categorized as medicines or drugs, and they have not received approval from the FDA for the prevention, treatment, or cure of any medical condition, ailment, or disease. We must emphasize that any form of bodily introduction of these products into humans or animals is strictly prohibited by law. It is essential to adhere to these guidelines to ensure compliance with legal and ethical standards in research and experimentation.