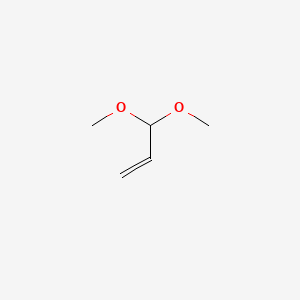
Acrolein dimethyl acetal
Overview
Description
Acrolein dimethyl acetal (3,3-dimethoxypropene, CAS 6044-68-4) is a protected form of acrolein, where the aldehyde group is masked as a dimethyl acetal. This compound is a colorless liquid with a boiling point of 89.5°C and a density of 0.9 g/cm³ . It is widely used in organic synthesis as a versatile three-carbon building block, particularly in Heck arylation reactions, pyridine synthesis, and the production of cinnamaldehydes and pharmaceutical intermediates . Its stability under neutral conditions and reactivity in acidic or catalytic environments make it indispensable in industrial chemistry.
Preparation Methods
Synthetic Routes and Reaction Conditions: Acrolein dimethyl acetal can be synthesized through the reaction of acrolein with methanol in the presence of an acid catalyst. The reaction typically proceeds as follows:
CH2=CHCHO+2CH3OH→CH2=CHCH(OCH3)2+H2O
This reaction is carried out under mild conditions, usually at room temperature, and requires careful control of the reaction environment to prevent side reactions .
Industrial Production Methods: In industrial settings, this compound is produced using a continuous flow process where acrolein and methanol are fed into a reactor containing an acid catalyst. The reaction mixture is then distilled to separate the desired product from by-products and unreacted starting materials .
Types of Reactions:
Common Reagents and Conditions:
Oxidation: Common oxidizing agents include potassium permanganate (KMnO4) and chromium trioxide (CrO3).
Reduction: Reducing agents such as lithium aluminum hydride (LiAlH4) and sodium borohydride (NaBH4) are typically used.
Substitution: Nucleophiles such as halides, amines, and thiols can be used in substitution reactions.
Major Products Formed:
Oxidation: Aldehydes and carboxylic acids.
Reduction: Alcohols.
Substitution: Various substituted derivatives depending on the nucleophile used.
Scientific Research Applications
Key Applications
- Chemical Synthesis
- Pharmaceuticals
- Agrochemicals
- Flavor and Fragrance Industry
Case Study 1: Catalytic Reactions
A study on the catalytic synthesis of acrolein diethyl acetal demonstrated the effectiveness of different catalysts in enhancing reaction yields. The research found that specific solid catalysts could significantly improve the efficiency of producing acetals from acrolein and alcohols .
Case Study 2: Environmental Impact
Research focusing on sustainable practices highlighted the potential for green chemistry applications involving this compound. Innovations in production methods aim to reduce environmental impact while maintaining efficiency, aligning with global sustainability goals .
Market Trends and Future Directions
The market for this compound is expected to grow due to increasing industrialization and demand for specialty chemicals. Key trends include:
- Sustainable Production : Companies are investing in eco-friendly production processes that utilize renewable raw materials.
- Technological Advancements : Ongoing research into advanced catalytic methods aims to enhance production efficiency and reduce costs associated with raw materials .
- Regulatory Compliance : Adhering to stringent environmental regulations remains a challenge but also presents opportunities for innovation.
Data Table: Market Insights
Year | Market Value (USD Billion) | Growth Rate (CAGR) |
---|---|---|
2023 | 1.95 | - |
2024 | 2.08 | - |
2030 | 3.05 | 6.56% |
Mechanism of Action
The mechanism of action of acrolein dimethyl acetal involves its high reactivity due to the presence of the acetal functional group. This reactivity allows it to participate in various chemical reactions, including nucleophilic addition and substitution . The compound can interact with cellular nucleophiles, particularly thiols, leading to changes in gene expression and cellular function . It can also deplete cellular glutathione, leading to oxidative stress and activation of transcription factors such as nuclear factor kappa B (NF-κB) and activator protein 1 (AP-1) .
Comparison with Similar Compounds
Acrolein Diethyl Acetal (CAS 3054-95-3)
- Synthesis: Prepared via the reaction of acrolein with ethanol and ethyl orthoformate using ammonium nitrate or p-toluenesulfonic acid, yielding up to 62% .
- Reactivity: Exhibits chemoselectivity in Heck reactions, producing ethyl 3-arylpropanoates or cinnamaldehyde derivatives, contrasting with the methyl esters formed from the dimethyl analog .
- Applications : Used in synthesizing natural products like (−)-laulimalide and microtubule-stabilizing agents .
- Stability : Hydrolyzes under acidic conditions (e.g., 0.1 M HCl at 40°C) to release acrolein, similar to the dimethyl variant but with slower kinetics due to larger ethoxy groups .
Crotonaldehyde Diethyl Acetal
- Reactivity : Less reactive than acrolein acetals in enzyme-catalyzed reactions due to steric hindrance from the methyl group .
- Product Selectivity: In Heck reactions, forms E-3-phenylcrotonaldehyde diethyl acetal and ethyl 3-phenylbutanoate, demonstrating divergent pathways compared to acrolein acetals .
4-Hydroxy-2E-nonenal (4-HNE) Dimethyl Acetal
- Synthesis : Derived via cross-metathesis between acrolein dimethyl acetal and octen-3-ol, highlighting the dimethyl acetal’s role in generating bioactive lipid derivatives .
- Stability : More resistant to hydrolysis than this compound, making it suitable for storage and controlled release in biological studies .
Tiglylaldehyde Diethyl Acetal (CAS 51786-74-4)
- Applications : Specialized in synthesizing branched aldehydes for fragrances, contrasting with this compound’s broader use in pharmaceuticals and agrochemicals .
Comparative Analysis Table
Key Research Findings
- Catalytic Pyridine Synthesis : this compound reacts with NH₃ over modified ZSM-5 zeolites to yield 3-picoline with enhanced selectivity compared to unmodified catalysts .
- Heck Reaction Chemoselectivity : The dimethyl acetal favors methyl ester formation (e.g., methyl 3-(4-nitrophenyl)propionate), while the diethyl analog produces ethyl esters or cinnamaldehydes .
- Stability in Biodiesel Production: this compound is a minor byproduct (4.5 wt.% in top column fractions) in biodiesel processing, indicating partial decomposition under industrial conditions .
Market and Industrial Relevance
The global market for this compound is projected to grow at a CAGR of 6.56%, reaching USD 3.05 billion by 2030, driven by demand in pharmaceuticals and specialty chemicals .
Biological Activity
Acrolein dimethyl acetal (DMA) is an acetal derivative of acrolein, a compound known for its significant biological activities. This article delves into the biological properties of this compound, exploring its synthesis, applications, and relevant case studies.
This compound has the chemical formula and is characterized by its relatively low toxicity compared to its parent compound, acrolein. The synthesis of this compound typically involves the reaction of acrolein with methanol in the presence of an acid catalyst. This process results in the formation of the acetal, which can be further utilized in various chemical reactions, including copolymerization.
Property | Value |
---|---|
Molecular Formula | C₅H₁₀O₂ |
Molecular Weight | 102.13 g/mol |
Boiling Point | 144 °C |
Density | 0.87 g/cm³ |
Solubility in Water | Slightly soluble |
Biological Activity
This compound exhibits a range of biological activities, primarily due to its conversion to acrolein upon hydrolysis. Acrolein itself is known for its antimicrobial, antifungal, and herbicidal properties.
- Antimicrobial Activity : Acrolein has been shown to effectively inhibit various bacterial strains including Escherichia coli and Pseudomonas putrefaciens. Studies indicate that acrolein and its derivatives can disrupt cellular membranes and inhibit enzyme activity, leading to microbial cell death .
- Antifungal Properties : Research indicates that acrolein is effective against fungal species such as Penicillium italicum and Saccharomyces cerevisiae. Its mechanism involves damaging fungal cell walls and membranes .
- Herbicidal Effects : Acrolein is recognized for controlling aquatic weeds and algae more effectively than traditional herbicides like chlorine. This property makes it a valuable agent in agricultural practices .
Case Studies
Several studies have explored the biological implications of this compound:
- Study on Antimicrobial Efficacy : A comparative study demonstrated that solutions containing acrolein derivatives exhibited significant reduction in bacterial counts in contaminated water samples. The effectiveness was attributed to the rapid release of acrolein from the dimethyl acetal under acidic conditions .
- Fungal Control in Agriculture : Field trials showed that applications of acrolein-based formulations significantly reduced fungal infections in crops compared to untreated controls. The results highlighted the potential use of this compound as a safe alternative to conventional fungicides .
- Polymerization Applications : this compound has also been utilized in palladium-catalyzed copolymerization reactions, producing branched copolymers with potential applications in drug delivery systems due to their biocompatibility and controlled release profiles .
Research Findings
Recent research emphasizes the versatility of this compound in various fields:
- Pharmaceutical Applications : Its role as a precursor in synthesizing bioactive compounds has been investigated, particularly in drug delivery systems where controlled release is critical .
- Environmental Impact : Studies suggest that due to its low toxicity and environmental persistence, using this compound could provide a more sustainable approach to pest control compared to traditional chemicals .
Q & A
Basic Research Questions
Q. What are the standard synthetic routes for preparing acrolein dimethyl acetal (ADMA) in laboratory settings?
ADMA is commonly synthesized via acid-catalyzed acetalization of acrolein with methanol . However, alternative methods include:
- Cross-metathesis reactions : ADMA reacts with octen-3-ol using a Grubbs catalyst to synthesize 4-hydroxy-2E-nonenal derivatives .
- Oxidative alkoxylation : In methanol, ADMA forms as an intermediate during the oxidation of acrolein, acting as a "buffer" compound to stabilize reactive intermediates .
Key considerations: Monitor reaction equilibrium (e.g., acrolein/methanol ratio) to avoid side products like acrolein methyl acetal.
Q. How can researchers characterize ADMA and its derivatives using analytical techniques?
- GC-MS : Use a polar capillary column (e.g., DB-WAX) to resolve ADMA (major ion m/z 71) from degradation products like 3-methoxypropanol (m/z 60) .
- NMR : Look for characteristic signals (e.g., δ 3.23 ppm for methoxy groups in H NMR) and δ 102–110 ppm for acetal carbons in C NMR .
- Density and boiling point : Confirm purity via physical properties (density: 0.862–0.887 g/mL at 25°C; boiling point: 89–90°C at atmospheric pressure) .
Q. What safety protocols are critical when handling ADMA in the lab?
- Storage : Stabilize ADMA in methanol to prevent dimerization or degradation .
- Exposure control : Use fume hoods and PPE (gloves, goggles) due to ADMA’s volatility and potential irritancy. Refer to Safety Data Sheets (SDS) for CAS 6044-68-4 .
Advanced Research Questions
Q. How does the choice of catalyst influence ADMA’s reactivity in copolymerization with ethene?
- Palladium-based catalysts : Cationic α-diimine Pd catalysts enable ADMA/ethene copolymerization to form branched copolymers. However, catalyst deactivation occurs via methanol elimination, forming inert η-1-methoxyallyl Pd species .
- Phosphine ligands : Tri-o-tolylphosphine enhances yields in Heck arylation reactions compared to triphenylphosphine, as demonstrated in aryl halide coupling with ADMA .
Optimization tip: Use excess alcohol to retard deactivation pathways .
Q. What mechanistic insights explain contradictory product distributions in ADMA-mediated reactions?
- Substituent effects : Electron-withdrawing groups (e.g., nitro in 4-nitrobromobenzene) favor ester formation (e.g., methyl 3-(4-nitrophenyl)propionate), while electron-donating groups (e.g., methoxy in 4-bromoanisole) yield mixed products (ester and acetal) due to competing pathways .
- Solvent dependence : In dioxane, ADMA forms hydrates instead of acetals, altering reaction outcomes .
Data reconciliation: Use kinetic studies (e.g., time-resolved GC-MS) to track intermediate formation.
Q. How can researchers mitigate ADMA’s instability during purification?
- Low-temperature distillation : Purify ADMA at reduced pressure (e.g., 0.1 mmHg) to minimize thermal decomposition .
- Inert atmosphere : Conduct purification under argon to prevent oxidation .
- Stabilizers : Add radical inhibitors (e.g., BHT) during storage .
Q. What role does ADMA play in natural product synthesis?
ADMA serves as a key intermediate in:
- Total synthesis of (-)-laulimalide : Used in stereoselective C–C bond formation via Pd-catalyzed coupling .
- 4-Hydroxy-2E-enals : Synthesized via cross-metathesis with unsaturated alcohols, enabling access to bioactive lipid derivatives .
Methodological note: Optimize reaction stoichiometry (1:1 ADMA:octen-3-ol) to maximize yield .
Q. Data Analysis and Reproducibility
Q. How should researchers address inconsistencies in copolymer molecular weight distributions?
- Catalyst batch variability : Pre-activate Pd catalysts with reducing agents (e.g., NaBH) to ensure consistent activity .
- GPC calibration : Use narrow polystyrene standards to accurately measure molecular weights.
Q. What strategies validate ADMA’s role as a “buffer” compound in oxidative alkoxylation?
- Isotopic labeling : Track O incorporation in ADMA during acrolein oxidation to confirm its intermediate role .
- Computational modeling : Simulate reaction pathways (e.g., DFT calculations) to identify transition states involving ADMA .
Properties
IUPAC Name |
3,3-dimethoxyprop-1-ene | |
---|---|---|
Source | PubChem | |
URL | https://pubchem.ncbi.nlm.nih.gov | |
Description | Data deposited in or computed by PubChem | |
InChI |
InChI=1S/C5H10O2/c1-4-5(6-2)7-3/h4-5H,1H2,2-3H3 | |
Source | PubChem | |
URL | https://pubchem.ncbi.nlm.nih.gov | |
Description | Data deposited in or computed by PubChem | |
InChI Key |
OBWGMYALGNDUNM-UHFFFAOYSA-N | |
Source | PubChem | |
URL | https://pubchem.ncbi.nlm.nih.gov | |
Description | Data deposited in or computed by PubChem | |
Canonical SMILES |
COC(C=C)OC | |
Source | PubChem | |
URL | https://pubchem.ncbi.nlm.nih.gov | |
Description | Data deposited in or computed by PubChem | |
Molecular Formula |
C5H10O2 | |
Source | PubChem | |
URL | https://pubchem.ncbi.nlm.nih.gov | |
Description | Data deposited in or computed by PubChem | |
DSSTOX Substance ID |
DTXSID30209185 | |
Record name | 1-Propene, 3,3-dimethoxy- | |
Source | EPA DSSTox | |
URL | https://comptox.epa.gov/dashboard/DTXSID30209185 | |
Description | DSSTox provides a high quality public chemistry resource for supporting improved predictive toxicology. | |
Molecular Weight |
102.13 g/mol | |
Source | PubChem | |
URL | https://pubchem.ncbi.nlm.nih.gov | |
Description | Data deposited in or computed by PubChem | |
Physical Description |
Colorless liquid with an unpleasant odor; [Alfa Aesar MSDS] | |
Record name | Acrolein dimethyl acetal | |
Source | Haz-Map, Information on Hazardous Chemicals and Occupational Diseases | |
URL | https://haz-map.com/Agents/20153 | |
Description | Haz-Map® is an occupational health database designed for health and safety professionals and for consumers seeking information about the adverse effects of workplace exposures to chemical and biological agents. | |
Explanation | Copyright (c) 2022 Haz-Map(R). All rights reserved. Unless otherwise indicated, all materials from Haz-Map are copyrighted by Haz-Map(R). No part of these materials, either text or image may be used for any purpose other than for personal use. Therefore, reproduction, modification, storage in a retrieval system or retransmission, in any form or by any means, electronic, mechanical or otherwise, for reasons other than personal use, is strictly prohibited without prior written permission. | |
Vapor Pressure |
85.0 [mmHg] | |
Record name | Acrolein dimethyl acetal | |
Source | Haz-Map, Information on Hazardous Chemicals and Occupational Diseases | |
URL | https://haz-map.com/Agents/20153 | |
Description | Haz-Map® is an occupational health database designed for health and safety professionals and for consumers seeking information about the adverse effects of workplace exposures to chemical and biological agents. | |
Explanation | Copyright (c) 2022 Haz-Map(R). All rights reserved. Unless otherwise indicated, all materials from Haz-Map are copyrighted by Haz-Map(R). No part of these materials, either text or image may be used for any purpose other than for personal use. Therefore, reproduction, modification, storage in a retrieval system or retransmission, in any form or by any means, electronic, mechanical or otherwise, for reasons other than personal use, is strictly prohibited without prior written permission. | |
CAS No. |
6044-68-4 | |
Record name | 3,3-Dimethoxy-1-propene | |
Source | CAS Common Chemistry | |
URL | https://commonchemistry.cas.org/detail?cas_rn=6044-68-4 | |
Description | CAS Common Chemistry is an open community resource for accessing chemical information. Nearly 500,000 chemical substances from CAS REGISTRY cover areas of community interest, including common and frequently regulated chemicals, and those relevant to high school and undergraduate chemistry classes. This chemical information, curated by our expert scientists, is provided in alignment with our mission as a division of the American Chemical Society. | |
Explanation | The data from CAS Common Chemistry is provided under a CC-BY-NC 4.0 license, unless otherwise stated. | |
Record name | 1-Propene, 3,3-dimethoxy- | |
Source | ChemIDplus | |
URL | https://pubchem.ncbi.nlm.nih.gov/substance/?source=chemidplus&sourceid=0006044684 | |
Description | ChemIDplus is a free, web search system that provides access to the structure and nomenclature authority files used for the identification of chemical substances cited in National Library of Medicine (NLM) databases, including the TOXNET system. | |
Record name | Acrolein dimethyl acetal | |
Source | DTP/NCI | |
URL | https://dtp.cancer.gov/dtpstandard/servlet/dwindex?searchtype=NSC&outputformat=html&searchlist=84156 | |
Description | The NCI Development Therapeutics Program (DTP) provides services and resources to the academic and private-sector research communities worldwide to facilitate the discovery and development of new cancer therapeutic agents. | |
Explanation | Unless otherwise indicated, all text within NCI products is free of copyright and may be reused without our permission. Credit the National Cancer Institute as the source. | |
Record name | 1-Propene, 3,3-dimethoxy- | |
Source | EPA DSSTox | |
URL | https://comptox.epa.gov/dashboard/DTXSID30209185 | |
Description | DSSTox provides a high quality public chemistry resource for supporting improved predictive toxicology. | |
Record name | 3,3-dimethoxypropene | |
Source | European Chemicals Agency (ECHA) | |
URL | https://echa.europa.eu/substance-information/-/substanceinfo/100.025.397 | |
Description | The European Chemicals Agency (ECHA) is an agency of the European Union which is the driving force among regulatory authorities in implementing the EU's groundbreaking chemicals legislation for the benefit of human health and the environment as well as for innovation and competitiveness. | |
Explanation | Use of the information, documents and data from the ECHA website is subject to the terms and conditions of this Legal Notice, and subject to other binding limitations provided for under applicable law, the information, documents and data made available on the ECHA website may be reproduced, distributed and/or used, totally or in part, for non-commercial purposes provided that ECHA is acknowledged as the source: "Source: European Chemicals Agency, http://echa.europa.eu/". Such acknowledgement must be included in each copy of the material. ECHA permits and encourages organisations and individuals to create links to the ECHA website under the following cumulative conditions: Links can only be made to webpages that provide a link to the Legal Notice page. | |
Retrosynthesis Analysis
AI-Powered Synthesis Planning: Our tool employs the Template_relevance Pistachio, Template_relevance Bkms_metabolic, Template_relevance Pistachio_ringbreaker, Template_relevance Reaxys, Template_relevance Reaxys_biocatalysis model, leveraging a vast database of chemical reactions to predict feasible synthetic routes.
One-Step Synthesis Focus: Specifically designed for one-step synthesis, it provides concise and direct routes for your target compounds, streamlining the synthesis process.
Accurate Predictions: Utilizing the extensive PISTACHIO, BKMS_METABOLIC, PISTACHIO_RINGBREAKER, REAXYS, REAXYS_BIOCATALYSIS database, our tool offers high-accuracy predictions, reflecting the latest in chemical research and data.
Strategy Settings
Precursor scoring | Relevance Heuristic |
---|---|
Min. plausibility | 0.01 |
Model | Template_relevance |
Template Set | Pistachio/Bkms_metabolic/Pistachio_ringbreaker/Reaxys/Reaxys_biocatalysis |
Top-N result to add to graph | 6 |
Feasible Synthetic Routes
Disclaimer and Information on In-Vitro Research Products
Please be aware that all articles and product information presented on BenchChem are intended solely for informational purposes. The products available for purchase on BenchChem are specifically designed for in-vitro studies, which are conducted outside of living organisms. In-vitro studies, derived from the Latin term "in glass," involve experiments performed in controlled laboratory settings using cells or tissues. It is important to note that these products are not categorized as medicines or drugs, and they have not received approval from the FDA for the prevention, treatment, or cure of any medical condition, ailment, or disease. We must emphasize that any form of bodily introduction of these products into humans or animals is strictly prohibited by law. It is essential to adhere to these guidelines to ensure compliance with legal and ethical standards in research and experimentation.