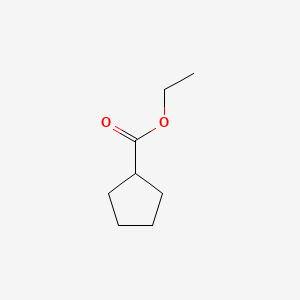
Ethyl cyclopentanecarboxylate
Overview
Description
Ethyl cyclopentanecarboxylate (CAS 5453-85-0) is a cyclic ester with the molecular formula C₈H₁₄O₂ and a molecular weight of 142.20 g/mol . Its structure consists of a cyclopentane ring fused to an ethyl ester group. This compound serves as a versatile intermediate in organic synthesis, particularly in the preparation of spirocyclic compounds, chiral building blocks, and pharmaceutical precursors .
Preparation Methods
Esterification of Cyclopentanecarboxylic Acid with Ethanol
The most common and classical method for preparing ethyl cyclopentanecarboxylate is the acid-catalyzed esterification of cyclopentanecarboxylic acid with ethanol. This method involves the following key steps:
- Reactants: Cyclopentanecarboxylic acid and ethanol.
- Catalyst: Typically a strong acid such as sulfuric acid or p-toluenesulfonic acid.
- Conditions: Reflux under removal of water to drive the equilibrium toward ester formation.
- Mechanism: Protonation of the carboxyl group enhances electrophilicity, allowing nucleophilic attack by ethanol, followed by dehydration to form the ester.
This method is well-documented and yields this compound with high purity and good yield. The reaction is reversible, so removal of water or use of excess ethanol is essential to maximize ester formation.
Parameter | Typical Value/Condition |
---|---|
Acid catalyst | H2SO4 or p-TsOH (catalytic) |
Temperature | Reflux (~78 °C for ethanol) |
Reaction time | Several hours (4–8 h) |
Molar ratio (acid:ethanol) | 1:1.5 to 1:3 |
Yield | Typically 70–90% |
This method is industrially favored due to its simplicity and scalability.
Base-Catalyzed Claisen Condensation and Subsequent Esterification
An alternative synthetic route involves the Claisen condensation of esters or related compounds to form cyclopentanecarboxylate derivatives, which can then be converted to this compound. The process includes:
- Step 1: Formation of enolate ions from esters using sodium ethoxide or sodium hydride.
- Step 2: Intramolecular condensation to form cyclic β-keto esters or diketones.
- Step 3: Hydrolysis and decarboxylation steps to yield cyclopentanecarboxylic acid derivatives.
- Step 4: Final esterification with ethanol under acidic conditions.
This method is more complex but allows for structural modifications and synthesis of substituted cyclopentanecarboxylates. It is supported by mechanistic studies showing enolate stabilization and nucleophilic attack on ester carbonyls.
Ring Contraction and Cyclization Approaches
Patented synthetic routes describe ring contraction methods starting from cyclohexane derivatives to obtain cyclopentanecarboxylic acid intermediates, which are then esterified to this compound. Key features include:
- Use of diketone intermediates formed by condensation reactions.
- Oxidation and hydrazone formation steps to facilitate ring contraction.
- Reaction with ethanol and acid catalysts to form the ester.
These methods are valuable for producing substituted cyclopentanecarboxylates and derivatives with specific functional groups.
Preparation via Diethyl Malonate and 1,4-Dibromobutane
A more recent method involves the synthesis of cyclopentanecarboxylic acid intermediates from diethyl malonate and 1,4-dibromobutane under basic conditions, followed by esterification:
- Step 1: Reaction of diethyl malonate with sodium ethoxide and 1,4-dibromobutane to form cyclopentane diester intermediates.
- Step 2: Hydrolysis and acidification to yield cyclopentanecarboxylic acid.
- Step 3: Esterification with ethanol to produce this compound.
This method offers advantages such as lower production costs, reduced environmental pollution, and shorter reaction times, making it attractive for industrial applications.
Hydrolysis and Reverse Reactions
This compound can undergo hydrolysis under acidic or basic conditions to regenerate cyclopentanecarboxylic acid and ethanol. Studies have shown:
- Acid-catalyzed hydrolysis proceeds via protonation of the carbonyl oxygen, enhancing electrophilicity.
- Base-promoted hydrolysis (saponification) involves nucleophilic attack by hydroxide ions.
- Hydrolysis rates vary depending on substituents and stereochemistry, with hydroxy-substituted derivatives hydrolyzing faster due to stereoelectronic effects.
Compound | Hydrolysis Rate (Relative) | Conditions |
---|---|---|
This compound | 1.0 (baseline) | 0.1 M NaOH, 25 °C |
Ethyl cis-2-hydroxycyclopentanecarboxylate | 1.8–11.0 | 0.1 M NaOH, 25 °C |
Ethyl trans-2-hydroxycyclopentanecarboxylate | 2.5–3.0 | 0.1 M NaOH, 25 °C |
This data is useful for understanding stability and reactivity during synthesis and purification.
Summary Table of Preparation Methods
Method | Key Reactants/Conditions | Advantages | Limitations |
---|---|---|---|
Acid-catalyzed esterification | Cyclopentanecarboxylic acid + ethanol + acid catalyst | Simple, high yield, scalable | Equilibrium reaction, requires water removal |
Claisen condensation + esterification | Esters + sodium ethoxide + acid catalyst | Allows structural modifications | Multi-step, requires careful control |
Ring contraction from cyclohexane derivatives | Diketones + hydrazine + acid catalyst | Access to substituted derivatives | Complex, patented process |
Diethyl malonate + 1,4-dibromobutane | Diethyl malonate + sodium ethoxide + 1,4-dibromobutane | Cost-effective, environmentally friendly | Requires multiple steps |
Research Findings and Notes
- The acid-catalyzed esterification remains the most widely used method due to its operational simplicity and efficiency.
- Claisen condensation routes provide versatility for synthesizing substituted esters but are less common industrially.
- Recent advances in ring contraction and malonate-based syntheses offer improved environmental profiles and cost benefits.
- Hydrolysis studies provide insight into reaction reversibility and stability, important for process optimization.
Chemical Reactions Analysis
Hydrolysis Reactions
Ethyl cyclopentanecarboxylate undergoes hydrolysis under acidic or basic conditions to yield cyclopentanecarboxylic acid and ethanol.
Mechanism :
-
Acid-catalyzed hydrolysis : Protonation of the carbonyl oxygen enhances electrophilicity, facilitating nucleophilic attack by water.
-
Base-promoted hydrolysis (saponification) : Hydroxide ion attacks the carbonyl carbon, forming a tetrahedral intermediate that collapses to release the carboxylate salt .
Experimental Findings :
-
A 1971 study compared hydrolysis rates of ethyl cis-2-hydroxycyclopentanecarboxylate (ECHC) and this compound (ECPC) in aqueous dioxane. ECHC hydrolyzed 1.8–11× faster than ECPC under basic conditions due to stereoelectronic effects .
-
Borate buffer accelerated ECHC hydrolysis by forming a borate-ester intermediate, suggesting potential catalytic applications .
Compound | Hydrolysis Rate (Relative to ECPC) | Conditions |
---|---|---|
ECPC | 1.0 (baseline) | 0.1 M NaOH, 25°C |
Ethyl cis-2-hydroxy-CC | 1.8–11.0 | 0.1 M NaOH, 25°C |
Ethyl trans-2-hydroxy-CC | 2.5–3.0 | 0.1 M NaOH, 25°C |
Reduction Reactions
The ester group is reducible to primary alcohols using strong hydride donors.
Reagents and Products :
-
Lithium aluminum hydride (LiAlH₄) : Converts ECPC to cyclopentylmethanol (C₆H₁₁CH₂OH) in anhydrous ether.
-
Sodium borohydride (NaBH₄) : Less effective due to the ester’s lower electrophilicity compared to ketones.
Industrial Application :
Reduced derivatives serve as intermediates in fragrance synthesis, leveraging the cyclopentane ring’s conformational rigidity.
Nucleophilic Substitution
The ester’s α-hydrogens participate in enolate formation, enabling alkylation and acylation.
Key Studies :
-
Enamine alkylation : Enamine derivatives of ECPC undergo γ-alkylation with electrophiles (e.g., allyl bromide), yielding substituted cyclopentane derivatives. This method achieved 70–85% yields in cyclic β-keto ester systems .
-
Acetoacetic ester synthesis : ECPC analogs react with alkyl halides to form α-alkylated products, decarboxylating to methyl ketones upon heating .
Example Reaction :
-
Enolate formation: ECPC + LDA → Enolate
-
Alkylation: Enolate + RX → α-Alkylated ester
-
Decarboxylation: Heat → Cyclopentyl methyl ketone
Oxidation and Functionalization
The cyclopentane ring and ester group allow selective oxidation:
Oxidation Pathways :
-
Ring oxidation : Ozonolysis or epoxidation modifies the cyclopentane structure.
-
Ester side-chain oxidation : Strong oxidants (e.g., KMnO₄) convert the ester to a carboxylic acid, though this is less common due to competing hydrolysis .
Patent Application :
WO2006045246A1 describes ECPC derivatives with hydroxymethyl or acetoxy groups for anti-inflammatory drug candidates, synthesized via controlled oxidation .
Scientific Research Applications
Ethyl cyclopentanecarboxylate has several applications in scientific research:
Biology: It serves as a building block for the synthesis of bioactive molecules and can be used in the study of enzyme-catalyzed reactions.
Medicine: It is involved in the development of new drugs and therapeutic agents.
Industry: It is used in the production of fragrances and flavoring agents due to its fruity odor.
Mechanism of Action
The mechanism of action of ethyl cyclopentanecarboxylate involves its interaction with various molecular targets and pathways. As an ester, it can undergo hydrolysis to release cyclopentanecarboxylic acid, which can then participate in further biochemical reactions. The specific pathways and targets depend on the context of its use, such as in drug development or chemical synthesis.
Comparison with Similar Compounds
Comparison with Structural Analogs
The following section compares ethyl cyclopentanecarboxylate with derivatives modified at the cyclopentane ring or ester group. Key differences in substituents, synthesis, and properties are highlighted.
Ketone Derivatives
Compound Name | Molecular Formula | Substituent(s) | Yield | Physical State | Key Applications |
---|---|---|---|---|---|
This compound | C₈H₁₄O₂ | None (parent compound) | N/A | Liquid/Oil | General synthesis intermediate |
Ethyl 2-oxocyclopentanecarboxylate | C₈H₁₂O₃ | 2-Oxo group | 61% | Oil | Spirocyclic α-proline synthesis |
Ethyl 3-oxocyclopentanecarboxylate | C₈H₁₂O₃ | 3-Oxo group | N/A | N/A | Chiral resolution studies |
Ethyl 2-oxo-1-((trifluoromethyl)thio)cyclopentanecarboxylate | C₉H₁₁F₃O₂S | 2-Oxo, 1-(SCF₃) | 39% | Pale-yellow oil | Fluorinated bioactive molecules |
Key Findings :
- The 2-oxo derivative (C₈H₁₂O₃) is synthesized via bromoacetonitrile addition, yielding 61% after flash chromatography . Its ketone group enhances reactivity for spiroannulation.
- The trifluoromethylthio analog (C₉H₁₁F₃O₂S) exhibits distinct NMR shifts (¹H: δ 4.44–4.14; ¹⁹F: δ −36.78) due to electron-withdrawing effects of the SCF₃ group .
Amino-Substituted Derivatives
Compound Name | Molecular Formula | Substituent(s) | Yield | Stereochemistry | Key Applications |
---|---|---|---|---|---|
Ethyl 2-(benzylamino)cyclopentanecarboxylate | C₁₅H₂₁NO₂ | 2-(Benzylamino) | 75% | cis/trans mixture | Chiral ligand synthesis |
Ethyl (1R,2S)-2-(4-fluorobenzylamino)cyclopentanecarboxylate | C₁₅H₂₀FNO₂ | 2-(4-Fluorobenzylamino) | 75% | Enantiopure (1R,2S) | Drug discovery building blocks |
Key Findings :
- Benzylamino derivatives (e.g., C₁₅H₂₁NO₂) are synthesized via nucleophilic substitution, achieving 75% yield. The benzyl group improves lipophilicity .
- Fluorinated analogs (e.g., C₁₅H₂₀FNO₂) show enhanced metabolic stability due to the fluorine atom, making them valuable in medicinal chemistry .
Hydroxy and Sulfonamido Derivatives
Compound Name | Molecular Formula | Substituent(s) | Yield | Physical State | Key Applications |
---|---|---|---|---|---|
Ethyl 1-(hydroxymethyl)cyclopentanecarboxylate | C₉H₁₆O₃ | 1-(Hydroxymethyl) | 97% | Solid | Polymer and dendrimer synthesis |
(±) Ethyl 2-hydroxy-3-(octylsulfonamido)cyclopentanecarboxylate | C₁₆H₂₉NO₅S | 2-Hydroxy, 3-sulfonamido | N/A | N/A | Enzyme inhibition studies |
Key Findings :
- The hydroxymethyl derivative (C₉H₁₆O₃) is a polar intermediate with a melting point suitable for crystallization .
- Sulfonamido analogs (e.g., C₁₆H₂₉NO₅S) exhibit hydrogen-bonding capabilities, relevant for targeting biological receptors .
Spirocyclic and Protective Group Derivatives
Compound Name | Molecular Formula | Substituent(s) | Yield | Key Feature |
---|---|---|---|---|
Ethyl 4-(tert-butyldimethylsilyloxy)-2-ethylcyclopentanecarboxylate | C₁₆H₃₂O₃Si | 4-TBDMS, 2-ethyl | 98% | Protective group stability |
Ethyl 2-azaspiro[4.4]nonan-1-one | C₁₀H₁₅NO₂ | Spirocyclic amine | 61% | Rigid scaffold |
Key Findings :
Biological Activity
Ethyl cyclopentanecarboxylate (ECC) is an organic compound with the molecular formula CHO and CAS number 5453-85-0. This compound is a type of ester derived from cyclopentanecarboxylic acid and is characterized by its unique cyclic structure, which influences its biological activity. This article provides a detailed overview of the biological activity of ECC, including its chemical properties, mechanisms of action, and relevant case studies.
The following table summarizes the key physical properties of this compound:
Property | Value |
---|---|
Molecular Weight | 142.2 g/mol |
Melting Point | 109 °C |
Boiling Point | 172-174 °C (at 752 Torr) |
Density | 0.9583 g/cm³ |
These properties suggest that ECC is a stable compound under standard conditions, making it suitable for various applications in organic synthesis and medicinal chemistry.
Mechanisms of Biological Activity
-
Hydrolysis and Metabolism :
ECC undergoes hydrolysis in aqueous environments, which can significantly affect its biological availability. Research indicates that the hydrolysis rate of ECC can be influenced by various factors, such as solvent composition and pH levels. For instance, it has been observed that the hydrolysis of ethyl cis-2-hydroxycyclopentanecarboxylate is significantly faster than that of ECC in sodium hydroxide solutions, suggesting potential implications for its bioactivity in different physiological conditions . -
Potential Therapeutic Applications :
The structural characteristics of ECC may allow it to interact with biological targets, including enzymes and receptors. Its ester functionality could facilitate interactions with biological membranes, potentially leading to various pharmacological effects. For instance, compounds similar to ECC have been studied for their roles in modulating enzyme activity and influencing metabolic pathways.
Study on Hydrolysis Rates
A study published in 1971 examined the hydrolysis kinetics of various cyclopentanecarboxylate esters, including ECC. The findings revealed that the rates of hydrolysis were significantly affected by the presence of different solvents, indicating that the biological activity of ECC could vary depending on environmental conditions .
Synthesis and Biological Evaluation
Research has also focused on synthesizing derivatives of ECC to evaluate their biological activities. For example, derivatives with additional functional groups have shown enhanced interactions with specific biological targets, suggesting that modifying the structure of ECC could lead to increased therapeutic efficacy .
Q & A
Q. Basic: What are the common synthetic routes for ethyl cyclopentanecarboxylate?
This compound is typically synthesized via esterification of cyclopentanecarboxylic acid with ethanol under acid catalysis or through Claisen condensation reactions. For example, in a base-catalyzed Claisen condensation, sodium ethoxide facilitates the deprotonation of this compound, leading to enolate formation and subsequent nucleophilic attack on another ester molecule. This method is detailed in mechanistic studies, where intermediates are stabilized by resonance . Alternative routes include ring-opening reactions of cyclic ketones followed by esterification, as demonstrated in patents using reagents like sodium nitrite and zinc under nitrogen atmospheres for derivatization .
Q. Basic: How is this compound characterized using NMR spectroscopy?
Key NMR signals for this compound derivatives include:
- 1H-NMR : Ethyl ester protons (δ ~4.15–4.28 ppm, q, J = 6.8–7.2 Hz), cyclopentane ring protons (δ ~1.26–2.50 ppm, multiplet patterns depending on substitution), and hydroxyl or amine protons (δ ~2.50–5.28 ppm, broad or split signals). For example, in (±)-ethyl 3-hydroxy-2-(octylsulfonamido)cyclopentanecarboxylate, the hydroxy proton appears at δ 5.28 ppm (d, J = 6.8 Hz), and ester protons at δ 4.18 ppm .
- 13C-NMR : Ester carbonyl (δ ~174 ppm), cyclopentane carbons (δ ~22–76 ppm). These peaks are critical for confirming ester functionality and ring substitution patterns .
Q. Advanced: How to resolve contradictions in NMR data when synthesizing derivatives?
Contradictions in NMR data often arise from stereochemical variations, solvent effects, or impurities. To address this:
- Comparative Analysis : Cross-reference with published spectra of analogous compounds. For instance, methyl 1-(methylamino)cyclopentanecarboxylate hydrochloride shows distinct aromatic proton signals (δ 7.48 ppm, d, J = 7.9 Hz) when para-toluenesulfonate is present, which can differentiate salt forms .
- Decoupling Experiments : Use 2D NMR (e.g., COSY, HSQC) to resolve overlapping signals. For example, in ethyl 2-hydroxy-3-(octylsulfonamido)cyclopentanecarboxylate, quintet splitting (δ 3.73 ppm) clarifies hydroxyl group positioning .
- Reproducibility Checks : Follow standardized protocols for sample preparation (e.g., drying, degassing) to minimize solvent artifacts .
Q. Advanced: What mechanisms are involved in the Claisen condensation of this compound?
The base-catalyzed Claisen condensation proceeds via:
Enolate Formation : Sodium ethoxide deprotonates the α-hydrogen, generating a resonance-stabilized enolate.
Nucleophilic Attack : The enolate attacks the carbonyl carbon of another ester molecule.
Elimination : A β-ketoester intermediate forms, releasing ethanol.
Key intermediates are stabilized by resonance, as shown in mechanistic studies where this compound undergoes cyclization to form six-membered transition states .
Q. Basic: What purification methods are effective for this compound derivatives?
- Column Chromatography : Use silica gel or aminosilica columns with hexane/ethyl acetate gradients (e.g., 30% ethyl acetate in hexanes) to separate polar derivatives .
- Recrystallization : For crystalline products, solvents like ethyl acetate or methanol are effective, as demonstrated in patents yielding >75% purity .
- Acid-Base Extraction : Remove unreacted starting materials by washing organic layers with aqueous NaHCO3 or dipotassium hydrogen phosphate .
Q. Advanced: How to optimize reaction conditions for high-yield synthesis?
- Temperature Control : Conduct reactions at 0°C for nitrosation steps to prevent side reactions, as shown in sodium nitrite-mediated syntheses .
- Catalyst Screening : Test Lewis acids (e.g., Zn) or organocatalysts to enhance enolate stability.
- Inert Atmospheres : Use nitrogen to prevent oxidation of sensitive intermediates, improving yields from 70% to 80% in multi-step reactions .
Q. Advanced: What strategies are used to handle stereochemical outcomes in cyclopentanecarboxylate derivatives?
- Chiral Auxiliaries : Employ enantiomerically pure reagents (e.g., tert-butyl (1R*,2S*,5R*)-2-hydroxy-5-...cyclopentanecarboxylate) to induce desired stereochemistry .
- Dynamic Resolution : Utilize kinetic resolution during crystallization or enzymatic catalysis to separate diastereomers.
- Computational Modeling : Predict steric effects using DFT calculations to guide substituent placement .
Q. Basic: What are the best practices for documenting synthesis procedures in academic research?
- Detailed Protocols : Include molar ratios, solvent volumes, and reaction times (e.g., "1.50 g, 7.75 mmol dissolved in 15 mL ethyl acetate") .
- Reproducibility : Provide spectral data (e.g., HRMS: m/z 426.2 [M+H]+) and purity metrics (e.g., HPLC retention time: 0.93 minutes) .
- Supplemental Data : Deposit extensive characterization data (e.g., >5 compounds) in supporting information to comply with journal guidelines .
Q. Advanced: How to analyze and interpret mass spectrometry data for cyclopentanecarboxylate compounds?
- High-Resolution MS (HRMS) : Confirm molecular formulas using exact mass (e.g., m/z 209.06703 [M+H]+ for C10H13N2OS) .
- Fragmentation Patterns : Identify key cleavage sites (e.g., ester bond breakage at m/z 156.181 for this compound) .
- Isotope Peaks : Use chlorine/bromine isotope signatures (if present) to validate structures .
Q. Advanced: How to design experiments to study the reactivity of this compound under varying conditions?
- Variable Screening : Test pH, temperature, and solvent polarity effects on reaction rates (e.g., acetic acid/water mixtures for nitrosation ).
- Kinetic Studies : Use in-situ FTIR or NMR to monitor enolate formation in real time.
- Cross-Coupling Reactions : Explore Suzuki-Miyaura reactions with boronate esters (e.g., ethyl (3Z)-3-[(4,4,5,5-tetramethyl-1,3,2-dioxaborolan-2-yl)methylene]cyclopentanecarboxylate) to functionalize the cyclopentane ring .
Properties
IUPAC Name |
ethyl cyclopentanecarboxylate | |
---|---|---|
Source | PubChem | |
URL | https://pubchem.ncbi.nlm.nih.gov | |
Description | Data deposited in or computed by PubChem | |
InChI |
InChI=1S/C8H14O2/c1-2-10-8(9)7-5-3-4-6-7/h7H,2-6H2,1H3 | |
Source | PubChem | |
URL | https://pubchem.ncbi.nlm.nih.gov | |
Description | Data deposited in or computed by PubChem | |
InChI Key |
UWSJCCUODNDXOT-UHFFFAOYSA-N | |
Source | PubChem | |
URL | https://pubchem.ncbi.nlm.nih.gov | |
Description | Data deposited in or computed by PubChem | |
Canonical SMILES |
CCOC(=O)C1CCCC1 | |
Source | PubChem | |
URL | https://pubchem.ncbi.nlm.nih.gov | |
Description | Data deposited in or computed by PubChem | |
Molecular Formula |
C8H14O2 | |
Source | PubChem | |
URL | https://pubchem.ncbi.nlm.nih.gov | |
Description | Data deposited in or computed by PubChem | |
DSSTOX Substance ID |
DTXSID60202942 | |
Record name | Ethyl cyclopentanecarboxylate | |
Source | EPA DSSTox | |
URL | https://comptox.epa.gov/dashboard/DTXSID60202942 | |
Description | DSSTox provides a high quality public chemistry resource for supporting improved predictive toxicology. | |
Molecular Weight |
142.20 g/mol | |
Source | PubChem | |
URL | https://pubchem.ncbi.nlm.nih.gov | |
Description | Data deposited in or computed by PubChem | |
CAS No. |
5453-85-0 | |
Record name | Ethyl cyclopentanecarboxylate | |
Source | CAS Common Chemistry | |
URL | https://commonchemistry.cas.org/detail?cas_rn=5453-85-0 | |
Description | CAS Common Chemistry is an open community resource for accessing chemical information. Nearly 500,000 chemical substances from CAS REGISTRY cover areas of community interest, including common and frequently regulated chemicals, and those relevant to high school and undergraduate chemistry classes. This chemical information, curated by our expert scientists, is provided in alignment with our mission as a division of the American Chemical Society. | |
Explanation | The data from CAS Common Chemistry is provided under a CC-BY-NC 4.0 license, unless otherwise stated. | |
Record name | Ethyl cyclopentanecarboxylate | |
Source | ChemIDplus | |
URL | https://pubchem.ncbi.nlm.nih.gov/substance/?source=chemidplus&sourceid=0005453850 | |
Description | ChemIDplus is a free, web search system that provides access to the structure and nomenclature authority files used for the identification of chemical substances cited in National Library of Medicine (NLM) databases, including the TOXNET system. | |
Record name | 5453-85-0 | |
Source | DTP/NCI | |
URL | https://dtp.cancer.gov/dtpstandard/servlet/dwindex?searchtype=NSC&outputformat=html&searchlist=18903 | |
Description | The NCI Development Therapeutics Program (DTP) provides services and resources to the academic and private-sector research communities worldwide to facilitate the discovery and development of new cancer therapeutic agents. | |
Explanation | Unless otherwise indicated, all text within NCI products is free of copyright and may be reused without our permission. Credit the National Cancer Institute as the source. | |
Record name | Ethyl cyclopentanecarboxylate | |
Source | EPA DSSTox | |
URL | https://comptox.epa.gov/dashboard/DTXSID60202942 | |
Description | DSSTox provides a high quality public chemistry resource for supporting improved predictive toxicology. | |
Record name | Ethyl cyclopentanecarboxylate | |
Source | European Chemicals Agency (ECHA) | |
URL | https://echa.europa.eu/substance-information/-/substanceinfo/100.024.272 | |
Description | The European Chemicals Agency (ECHA) is an agency of the European Union which is the driving force among regulatory authorities in implementing the EU's groundbreaking chemicals legislation for the benefit of human health and the environment as well as for innovation and competitiveness. | |
Explanation | Use of the information, documents and data from the ECHA website is subject to the terms and conditions of this Legal Notice, and subject to other binding limitations provided for under applicable law, the information, documents and data made available on the ECHA website may be reproduced, distributed and/or used, totally or in part, for non-commercial purposes provided that ECHA is acknowledged as the source: "Source: European Chemicals Agency, http://echa.europa.eu/". Such acknowledgement must be included in each copy of the material. ECHA permits and encourages organisations and individuals to create links to the ECHA website under the following cumulative conditions: Links can only be made to webpages that provide a link to the Legal Notice page. | |
Record name | ETHYL CYCLOPENTANECARBOXYLATE | |
Source | FDA Global Substance Registration System (GSRS) | |
URL | https://gsrs.ncats.nih.gov/ginas/app/beta/substances/2KB7W3C29Q | |
Description | The FDA Global Substance Registration System (GSRS) enables the efficient and accurate exchange of information on what substances are in regulated products. Instead of relying on names, which vary across regulatory domains, countries, and regions, the GSRS knowledge base makes it possible for substances to be defined by standardized, scientific descriptions. | |
Explanation | Unless otherwise noted, the contents of the FDA website (www.fda.gov), both text and graphics, are not copyrighted. They are in the public domain and may be republished, reprinted and otherwise used freely by anyone without the need to obtain permission from FDA. Credit to the U.S. Food and Drug Administration as the source is appreciated but not required. | |
Synthesis routes and methods I
Procedure details
Synthesis routes and methods II
Procedure details
Retrosynthesis Analysis
AI-Powered Synthesis Planning: Our tool employs the Template_relevance Pistachio, Template_relevance Bkms_metabolic, Template_relevance Pistachio_ringbreaker, Template_relevance Reaxys, Template_relevance Reaxys_biocatalysis model, leveraging a vast database of chemical reactions to predict feasible synthetic routes.
One-Step Synthesis Focus: Specifically designed for one-step synthesis, it provides concise and direct routes for your target compounds, streamlining the synthesis process.
Accurate Predictions: Utilizing the extensive PISTACHIO, BKMS_METABOLIC, PISTACHIO_RINGBREAKER, REAXYS, REAXYS_BIOCATALYSIS database, our tool offers high-accuracy predictions, reflecting the latest in chemical research and data.
Strategy Settings
Precursor scoring | Relevance Heuristic |
---|---|
Min. plausibility | 0.01 |
Model | Template_relevance |
Template Set | Pistachio/Bkms_metabolic/Pistachio_ringbreaker/Reaxys/Reaxys_biocatalysis |
Top-N result to add to graph | 6 |
Feasible Synthetic Routes
Disclaimer and Information on In-Vitro Research Products
Please be aware that all articles and product information presented on BenchChem are intended solely for informational purposes. The products available for purchase on BenchChem are specifically designed for in-vitro studies, which are conducted outside of living organisms. In-vitro studies, derived from the Latin term "in glass," involve experiments performed in controlled laboratory settings using cells or tissues. It is important to note that these products are not categorized as medicines or drugs, and they have not received approval from the FDA for the prevention, treatment, or cure of any medical condition, ailment, or disease. We must emphasize that any form of bodily introduction of these products into humans or animals is strictly prohibited by law. It is essential to adhere to these guidelines to ensure compliance with legal and ethical standards in research and experimentation.