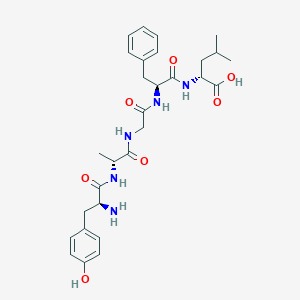
Dadle
Overview
Description
- DADLE is a synthetic opioid peptide with analgesic properties.
- Its peptide sequence is Tyr-D-Ala-Gly-Phe-D-Leu.
- While it is often considered a selective δ-opioid receptor agonist, it also binds to the μ1 subtype of μ-opioid receptors .
Mechanism of Action
Target of Action
DADLE, or [D-Ala2, D-Leu5]-Enkephalin, is a synthetic peptide and a highly selective agonist for the delta opioid receptor (δOR) . The δOR is expressed on brain microvascular endothelial cells (BMECs), and this compound’s interaction with these receptors plays a crucial role in its mechanism of action .
Mode of Action
This compound interacts with δORs on BMECs, leading to a series of cellular changes . This interaction is thought to be responsible for this compound’s neuroprotective effects, as it has been shown to reduce apoptosis and promote neuronal survival . The protective effects of this compound can be blocked by opioid antagonists, indicating that its action is mediated through opioid receptors .
Biochemical Pathways
This compound’s interaction with δORs triggers the AMPK/mTOR/ULK1 signaling pathway . This pathway plays a crucial role in autophagy, a cellular process involved in the degradation and recycling of cellular components. By activating this pathway, this compound enhances autophagy, which is thought to contribute to its neuroprotective effects .
Pharmacokinetics
It is known that this compound is a small molecule, which suggests it may have good bioavailability .
Result of Action
This compound’s action results in a number of molecular and cellular effects. It has been shown to decrease apoptosis and promote neuronal survival . In addition, this compound enhances autophagic flux and inhibits necroptosis, a form of programmed cell death . These effects are thought to contribute to this compound’s neuroprotective properties.
Action Environment
Environmental factors can influence the action, efficacy, and stability of this compound. For example, this compound has been shown to induce a hibernation-like state in non-hibernating species, suggesting that it may be influenced by environmental conditions . .
Biochemical Analysis
Biochemical Properties
“Dadle” interacts with delta opioid receptors, stimulating extracellular signal-regulated protein kinases (ERK), which are members of the mitogen-activated protein kinases (MAPK) . These interactions play a key role in neuronal protection against hypoxic and ischemic conditions .
Cellular Effects
“this compound” exerts protective effects on various types of cells, particularly neurons, under hypoxic and ischemic conditions . It influences cell function by attenuating apoptosis via the MEK/ERK antiapoptotic pathway .
Molecular Mechanism
“this compound” exerts its effects at the molecular level through binding interactions with delta opioid receptors . It activates the MEK-ERK pathway, leading to decreased apoptosis and improved neuronal survival .
Temporal Effects in Laboratory Settings
“this compound” has shown to provide dose-dependent protection on spinal cord ischemia-reperfusion (I/R) injury in rabbits . Over time, it reduces the rate of paraplegia and increases the number of surviving neurons .
Dosage Effects in Animal Models
In animal models, the effects of “this compound” vary with different dosages . At a dosage of 0.05 mg/kg, “this compound” significantly reduces serum malondialdehyde (MDA) and nitric oxide (NO) levels, while increasing glutathione peroxidase (GSH-Px) and superoxide dismutase (SOD) levels .
Metabolic Pathways
“this compound” is involved in the MEK-ERK pathway . It interacts with delta opioid receptors and stimulates ERK, a member of the MAPK .
Preparation Methods
- DADLE can be synthesized using various routes, including solid-phase peptide synthesis.
- Industrial production methods may involve large-scale peptide synthesis or recombinant expression in microorganisms.
Chemical Reactions Analysis
- DADLE undergoes various reactions, including oxidation, reduction, and substitution.
- Common reagents include protecting groups for amino acids during synthesis.
- Major products formed depend on the specific reaction conditions.
Scientific Research Applications
- In chemistry: DADLE serves as a model peptide for studying opioid receptor interactions.
- In biology: It helps elucidate opioid receptor signaling pathways.
- In medicine: Research explores its potential as an analgesic and neuroprotective agent.
- In industry: this compound’s applications are limited due to its peptide nature.
Comparison with Similar Compounds
- DADLE’s uniqueness lies in its specific sequence and receptor selectivity.
- Similar compounds include other enkephalins (e.g., DAMGO) and endogenous opioid peptides.
Biological Activity
DADLE, or [D-Ala2, D-Leu5]-enkephalin, is a synthetic peptide that acts primarily as a delta-opioid receptor (DOR) agonist. Its biological activity has been extensively studied across various systems, demonstrating protective effects in multiple organ systems and potential therapeutic applications. This article synthesizes findings from diverse research studies, highlighting this compound's mechanisms of action, effects on cellular proliferation, and implications for organ protection.
This compound exerts its biological effects primarily through its interaction with delta-opioid receptors. The following mechanisms have been identified:
- Neuroprotection : this compound has been shown to enhance the survival of dopaminergic neurons and protect against neurotoxic damage. For instance, it significantly reduced the methamphetamine-induced loss of dopaminergic terminals in mouse models .
- Cardioprotection : In studies involving myocardial ischemia/reperfusion (I/R) injury, this compound was found to suppress the expression of apoptotic markers such as caspase-3 and β-catenin, thereby mitigating heart tissue damage .
- Hepatic Protection : Research indicates that this compound improves liver function post-I/R injury through activation of the Nrf2/HO-1 pathway, suggesting a role in oxidative stress response .
Table 1: Summary of Biological Activities of this compound
Effects on Cellular Proliferation
This compound's impact on cellular proliferation has been notably characterized in studies using AF5 dopaminergic progenitor cells. The compound demonstrated significant antiproliferative effects:
- Growth Inhibition : this compound at concentrations ranging from 1 pM to 10 nM inhibited cellular proliferation, particularly evident at the 120-hour mark. This effect was more pronounced when combined with naltrexone, an opioid antagonist .
- Cell Cycle Arrest : Flow cytometry analysis revealed that this compound treatment led to a significant increase in cells arrested at the G1 phase of the cell cycle. This suggests that this compound may influence cell cycle dynamics as part of its antiproliferative action .
Table 2: Effects of this compound on AF5 Cell Cycle Progression
Treatment | G1 Phase (%) | S Phase (%) | G2/M Phase (%) |
---|---|---|---|
Control | 52 | 46 | 2.48 |
This compound | 68.43 | 36.77 | 0.8 |
Naltrexone | 61.23 | 38.77 | 0 |
This compound + Naltrexone | 70.12 | 30.88 | 0 |
Case Studies and Research Findings
Several case studies have underscored the therapeutic potential of this compound:
- Myocardial Ischemia/Reperfusion Injury :
- Hepatic Ischemia/Reperfusion Injury :
- Neuroprotection in Dopaminergic Systems :
Properties
IUPAC Name |
(2R)-2-[[(2S)-2-[[2-[[(2R)-2-[[(2S)-2-amino-3-(4-hydroxyphenyl)propanoyl]amino]propanoyl]amino]acetyl]amino]-3-phenylpropanoyl]amino]-4-methylpentanoic acid | |
---|---|---|
Source | PubChem | |
URL | https://pubchem.ncbi.nlm.nih.gov | |
Description | Data deposited in or computed by PubChem | |
InChI |
InChI=1S/C29H39N5O7/c1-17(2)13-24(29(40)41)34-28(39)23(15-19-7-5-4-6-8-19)33-25(36)16-31-26(37)18(3)32-27(38)22(30)14-20-9-11-21(35)12-10-20/h4-12,17-18,22-24,35H,13-16,30H2,1-3H3,(H,31,37)(H,32,38)(H,33,36)(H,34,39)(H,40,41)/t18-,22+,23+,24-/m1/s1 | |
Source | PubChem | |
URL | https://pubchem.ncbi.nlm.nih.gov | |
Description | Data deposited in or computed by PubChem | |
InChI Key |
ZHUJMSMQIPIPTF-IBURTVSXSA-N | |
Source | PubChem | |
URL | https://pubchem.ncbi.nlm.nih.gov | |
Description | Data deposited in or computed by PubChem | |
Canonical SMILES |
CC(C)CC(C(=O)O)NC(=O)C(CC1=CC=CC=C1)NC(=O)CNC(=O)C(C)NC(=O)C(CC2=CC=C(C=C2)O)N | |
Source | PubChem | |
URL | https://pubchem.ncbi.nlm.nih.gov | |
Description | Data deposited in or computed by PubChem | |
Isomeric SMILES |
C[C@H](C(=O)NCC(=O)N[C@@H](CC1=CC=CC=C1)C(=O)N[C@H](CC(C)C)C(=O)O)NC(=O)[C@H](CC2=CC=C(C=C2)O)N | |
Source | PubChem | |
URL | https://pubchem.ncbi.nlm.nih.gov | |
Description | Data deposited in or computed by PubChem | |
Molecular Formula |
C29H39N5O7 | |
Source | PubChem | |
URL | https://pubchem.ncbi.nlm.nih.gov | |
Description | Data deposited in or computed by PubChem | |
Molecular Weight |
569.6 g/mol | |
Source | PubChem | |
URL | https://pubchem.ncbi.nlm.nih.gov | |
Description | Data deposited in or computed by PubChem | |
CAS No. |
63631-40-3 | |
Record name | Enkephalin, leucine-2-alanine | |
Source | ChemIDplus | |
URL | https://pubchem.ncbi.nlm.nih.gov/substance/?source=chemidplus&sourceid=0063631403 | |
Description | ChemIDplus is a free, web search system that provides access to the structure and nomenclature authority files used for the identification of chemical substances cited in National Library of Medicine (NLM) databases, including the TOXNET system. | |
Record name | DADLE | |
Source | DrugBank | |
URL | https://www.drugbank.ca/drugs/DB08856 | |
Description | The DrugBank database is a unique bioinformatics and cheminformatics resource that combines detailed drug (i.e. chemical, pharmacological and pharmaceutical) data with comprehensive drug target (i.e. sequence, structure, and pathway) information. | |
Explanation | Creative Common's Attribution-NonCommercial 4.0 International License (http://creativecommons.org/licenses/by-nc/4.0/legalcode) | |
Record name | ENKEPHALIN-(2-D-ALA, 5-D-LEU) | |
Source | FDA Global Substance Registration System (GSRS) | |
URL | https://gsrs.ncats.nih.gov/ginas/app/beta/substances/HB4QD9GL6F | |
Description | The FDA Global Substance Registration System (GSRS) enables the efficient and accurate exchange of information on what substances are in regulated products. Instead of relying on names, which vary across regulatory domains, countries, and regions, the GSRS knowledge base makes it possible for substances to be defined by standardized, scientific descriptions. | |
Explanation | Unless otherwise noted, the contents of the FDA website (www.fda.gov), both text and graphics, are not copyrighted. They are in the public domain and may be republished, reprinted and otherwise used freely by anyone without the need to obtain permission from FDA. Credit to the U.S. Food and Drug Administration as the source is appreciated but not required. | |
Retrosynthesis Analysis
AI-Powered Synthesis Planning: Our tool employs the Template_relevance Pistachio, Template_relevance Bkms_metabolic, Template_relevance Pistachio_ringbreaker, Template_relevance Reaxys, Template_relevance Reaxys_biocatalysis model, leveraging a vast database of chemical reactions to predict feasible synthetic routes.
One-Step Synthesis Focus: Specifically designed for one-step synthesis, it provides concise and direct routes for your target compounds, streamlining the synthesis process.
Accurate Predictions: Utilizing the extensive PISTACHIO, BKMS_METABOLIC, PISTACHIO_RINGBREAKER, REAXYS, REAXYS_BIOCATALYSIS database, our tool offers high-accuracy predictions, reflecting the latest in chemical research and data.
Strategy Settings
Precursor scoring | Relevance Heuristic |
---|---|
Min. plausibility | 0.01 |
Model | Template_relevance |
Template Set | Pistachio/Bkms_metabolic/Pistachio_ringbreaker/Reaxys/Reaxys_biocatalysis |
Top-N result to add to graph | 6 |
Feasible Synthetic Routes
Q1: What is the primary molecular target of DADLE?
A1: this compound acts primarily as a selective agonist of the delta opioid receptor (DOR), a G protein-coupled receptor. [, , , , , , , ]
Q2: How does this compound binding to DOR elicit its effects?
A2: this compound binding to DOR initiates signaling cascades primarily through Gi/Go proteins, leading to inhibition of adenylyl cyclase and a decrease in cyclic AMP (cAMP) levels. [, ] This, in turn, influences various downstream pathways involved in pain modulation, neuroprotection, and other physiological processes. [, , ]
Q3: Does this compound interact with other opioid receptors?
A3: While this compound displays selectivity for DOR, studies indicate it can also interact with mu opioid receptors (MOR) at higher concentrations, albeit with lower affinity compared to DOR. [, ] This interaction contributes to its complex pharmacological profile.
Q4: How does this compound's interaction with MOR differ from its interaction with DOR?
A4: this compound's interaction with MOR can activate distinct downstream signaling pathways compared to DOR, potentially leading to different physiological effects. [] For instance, studies suggest that this compound's cytotoxic effects at high concentrations might be mediated through MOR activation and subsequent Fas ligand (FasL) upregulation. []
Q5: What is the role of receptor endocytosis in this compound's activity?
A5: Research indicates that DOR endocytosis is crucial for this compound's analgesic effects. [] Prolonged exposure to high this compound concentrations can induce DOR-dependent endocytosis and downstream signaling involving protein kinase C (PKC), potentially contributing to neuronal hyperexcitability. []
Q6: How does this compound affect neuronal survival and apoptosis?
A6: this compound exhibits neuroprotective properties by activating pro-survival kinases like Akt and extracellular signal-regulated kinase (ERK) via epidermal growth factor receptor (EGFR) transactivation. [] It also influences apoptosis by modulating the balance of pro-apoptotic (e.g., Bax) and anti-apoptotic (e.g., Bcl-2) proteins in various cell types, including neurons and astrocytes. [, , ]
Q7: What is the molecular formula and weight of this compound?
A7: this compound (C30H42N6O7) has a molecular weight of 598.7 g/mol.
Q8: How do structural modifications of this compound impact its activity?
A8: Modifications to this compound's structure can significantly alter its pharmacological properties, including potency, selectivity, and metabolic stability. [, , ] For instance, capping the N- and C-termini or cyclizing the peptide with a coumarinic acid linker aimed to improve its metabolic stability and bioavailability. [, , , ]
Q9: How is this compound metabolized in the body?
A9: this compound is primarily metabolized by enzymatic degradation, with endopeptidases playing a major role in its elimination. [] Its cyclic prodrugs, designed to enhance stability, undergo esterase-catalyzed bioconversion to release this compound. [, ]
Q10: What are the primary routes of this compound elimination?
A10: this compound is primarily eliminated through metabolism, while its cyclic prodrugs are mainly excreted through the biliary route. [, ]
Q11: What factors limit this compound's permeation across biological barriers?
A11: this compound's permeation across biological barriers, such as the blood-brain barrier (BBB) and intestinal mucosa, is restricted by efflux transporters like P-glycoprotein (P-gp) and metabolic enzymes, particularly CYP450 enzymes. [, , ]
Q12: How do the pharmacokinetic properties of this compound differ across species?
A12: Significant species differences exist in the disposition of this compound and its cyclic prodrugs. [] For instance, brain uptake of the cyclic prodrug OMCA-DADLE and this compound in guinea pigs was significantly higher compared to rats. [] This highlights the importance of considering species-specific factors when interpreting preclinical data.
Q13: What in vitro models have been used to study this compound's effects?
A13: Various in vitro models, including isolated organ preparations (e.g., rat heart, guinea pig ileum), primary cell cultures (e.g., rat cortical neurons, astrocytes), and immortalized cell lines (e.g., NG108-15, PC12, HepG2, AF5, U937) have been utilized to investigate this compound's effects on various cellular processes. [, , , , , , , , , , , , , , ]
Q14: What in vivo models have been used to study this compound's therapeutic potential?
A14: Animal models of various conditions, including global cerebral ischemia-reperfusion injury in rats, spinal cord injury in mice, sepsis in rats, hemorrhagic shock in rats, and pulmonary ischemia-reperfusion injury in pigs, have been employed to evaluate the therapeutic potential of this compound. [, , , , , , , , , ]
Q15: What is known about the long-term effects of this compound?
A15: Further research is needed to fully elucidate the long-term effects of this compound. Current knowledge primarily stems from preclinical studies, highlighting the need for more comprehensive investigations, particularly in the context of chronic administration.
Q16: What drug delivery strategies have been explored to enhance this compound's therapeutic efficacy?
A16: Strategies to improve this compound's delivery and targeting include the development of cyclic prodrugs to enhance stability and potentially facilitate transport across biological barriers. [, , , ] Intrathecal administration has also been investigated for targeted delivery to the spinal cord. [, ]
Q17: How does this compound relate to the phenomenon of hibernation?
A17: this compound has been identified as a potential hibernation-inducing agent. [, ] Research suggests that its ability to induce a hypometabolic state similar to hibernation may contribute to its protective effects in various organs, including the brain.
Q18: What are the implications of this compound's effects on KATP channels?
A18: this compound's ability to open ATP-sensitive potassium (KATP) channels is thought to be a key mechanism underlying its protective effects against ischemia-reperfusion injury in various organs. [, ] This mechanism contributes to its potential therapeutic value in conditions like myocardial infarction and stroke.
Q19: What is the historical context of this compound research?
A19: this compound's discovery and subsequent research stem from efforts to understand the endogenous opioid system and explore its therapeutic potential. [] Over the years, research has uncovered its diverse pharmacological actions, fueling continued interest in its possible applications.
Disclaimer and Information on In-Vitro Research Products
Please be aware that all articles and product information presented on BenchChem are intended solely for informational purposes. The products available for purchase on BenchChem are specifically designed for in-vitro studies, which are conducted outside of living organisms. In-vitro studies, derived from the Latin term "in glass," involve experiments performed in controlled laboratory settings using cells or tissues. It is important to note that these products are not categorized as medicines or drugs, and they have not received approval from the FDA for the prevention, treatment, or cure of any medical condition, ailment, or disease. We must emphasize that any form of bodily introduction of these products into humans or animals is strictly prohibited by law. It is essential to adhere to these guidelines to ensure compliance with legal and ethical standards in research and experimentation.