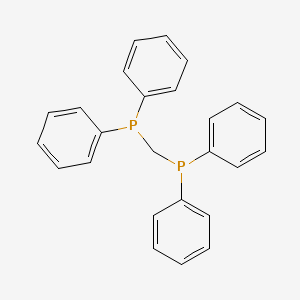
Bis(diphenylphosphino)methane
Overview
Description
Bis(diphenylphosphino)methane, also known as methylenebis(diphenylphosphine), is an organophosphorus compound with the chemical formula CH₂(PPh₂)₂. This compound is a white crystalline powder and is widely used in inorganic and organometallic chemistry as a ligand. It is particularly known for its ability to form chelating complexes with metals, which makes it valuable in various chemical reactions and industrial applications .
Mechanism of Action
Target of Action
Bis(diphenylphosphino)methane (DPPM) is an organophosphorus compound that primarily targets metals . It acts as a chelating ligand, meaning it can bond to metals with two phosphorus donor atoms .
Mode of Action
DPPM interacts with its targets by forming a four-membered ring with the constituents MP2C . This interaction promotes the formation of bimetallic complexes that feature five-membered M2P2C rings . The natural bite angle of DPPM is 73° .
Biochemical Pathways
The biochemical pathways affected by DPPM are primarily related to the formation of bimetallic complexes . The formation of these complexes can have downstream effects on various biochemical processes, particularly those involving metal ions.
Pharmacokinetics
It is known that dppm is a white, crystalline powder that is insoluble in water
Result of Action
The primary result of DPPM’s action is the formation of bimetallic complexes . These complexes can have various molecular and cellular effects, depending on the specific metals involved and the environmental context.
Action Environment
The action, efficacy, and stability of DPPM can be influenced by various environmental factors. For example, its ability to form complexes may be affected by the presence and concentration of specific metal ions. Additionally, as DPPM is air sensitive , its stability and reactivity may be influenced by exposure to air.
Preparation Methods
Bis(diphenylphosphino)methane can be synthesized through several methods. One common synthetic route involves the reaction of sodium diphenylphosphide with dichloromethane. The reaction proceeds as follows :
[ \text{Ph}_3\text{P} + 2 \text{Na} \rightarrow \text{Ph}_2\text{PNa} + \text{NaPh} ] [ 2 \text{NaPPh}_2 + \text{CH}_2\text{Cl}_2 \rightarrow \text{Ph}_2\text{PCH}_2\text{PPh}_2 + 2 \text{NaCl} ]
In industrial settings, the production of this compound may involve similar reactions but on a larger scale, with careful control of reaction conditions to ensure high yield and purity.
Chemical Reactions Analysis
Bis(diphenylphosphino)methane undergoes various chemical reactions, including oxidation, reduction, and substitution reactions. Some common reactions include:
Oxidation: The ligand can be oxidized to form the corresponding oxides and sulfides, such as CH₂[P(E)Ph₂]₂ (E = O, S).
Reduction: The methylene group in this compound is mildly acidic and can be deprotonated to form diphosphinomethanide derivatives.
Substitution: The compound can participate in substitution reactions to form various coordination complexes with metals.
Common reagents used in these reactions include oxidizing agents like oxygen or sulfur, and bases for deprotonation. The major products formed from these reactions are typically metal complexes, which are valuable in catalysis and other applications.
Scientific Research Applications
Bis(diphenylphosphino)methane has a wide range of applications in scientific research:
Chemistry: It is used as a ligand in the synthesis of coordination compounds and metal complexes.
Biology: The compound’s metal complexes are studied for their potential biological activities and interactions with biomolecules.
Medicine: Research is ongoing to explore the potential medicinal applications of this compound complexes, particularly in drug delivery and imaging.
Comparison with Similar Compounds
Bis(diphenylphosphino)methane can be compared with other similar compounds, such as:
1,2-Bis(diphenylphosphino)ethane (dppe): This compound has a similar structure but with an ethylene bridge instead of a methylene bridge. It forms different coordination complexes and has distinct reactivity.
Bis(ditertiaryphosphino)thioethers: These compounds have a sulfur atom replacing the methylene backbone, which alters their stability and electronic properties.
1,1-Bis(diphenylphosphino)ethane (dppm): This compound is similar but has different substituents on the phosphorus atoms, leading to variations in its coordination chemistry and applications.
This compound is unique due to its specific bite angle and the stability of its metal complexes, making it particularly valuable in catalysis and coordination chemistry.
Biological Activity
Introduction
Bis(diphenylphosphino)methane (dppm) is a diphosphine ligand widely studied for its coordination chemistry and biological activities. This article explores the biological activity of dppm, particularly its anti-cancer properties, proteasome inhibition, and potential therapeutic applications. The findings are supported by various research studies, case studies, and detailed data tables.
Dppm is characterized by its unique structure, which consists of two diphenylphosphino groups connected by a methylene bridge. This configuration allows dppm to form stable complexes with various metals, enhancing its biological activity.
Table 1: Structural Characteristics of dppm
Property | Value |
---|---|
Molecular Formula | C18H18P2 |
Molecular Weight | 306.31 g/mol |
Ligand Type | Diphosphine |
Coordination Modes | Bidentate |
Anti-Cancer Activity
Research has demonstrated that dppm exhibits significant anti-cancer properties through mechanisms such as proteasome inhibition. A study highlighted its effectiveness in inducing apoptosis in breast cancer cells (MDA-MB-231) by inhibiting the 26S proteasome, which is essential for degrading damaged proteins.
Case Study: Proteasome Inhibition
In vitro experiments showed that complexes formed with dppm significantly inhibited proteasomal chymotrypsin-like activity. For instance, a complex containing dppm demonstrated approximately 80% inhibition at a concentration of 6 μM, leading to increased levels of ubiquitinated proteins and apoptosis markers such as cleaved PARP and activated caspase-3.
Table 2: Effects of dppm on Proteasome Activity
Concentration (μM) | Inhibition (%) | Apoptosis Induction (Caspase-3 Activity Increase %) |
---|---|---|
1 | 10 | 30 |
3 | 35 | 60 |
6 | 80 | 180 |
The mechanism underlying the anti-cancer effects of dppm involves the disruption of the ubiquitin-proteasome pathway. By inhibiting proteasomal activity, dppm leads to the accumulation of pro-apoptotic factors and the induction of cell death in cancer cells.
Other Biological Activities
Beyond its anti-cancer properties, dppm has been investigated for its role in other biological contexts:
- Metal Complexes : Dppm forms various metal complexes that exhibit antibacterial and antifungal activities.
- Enzyme Inhibition : Studies have indicated that dppm can inhibit certain enzymes involved in cancer metabolism.
- Neuroprotective Effects : Preliminary research suggests potential neuroprotective effects, although further studies are needed to confirm these findings.
Table 3: Summary of Biological Activities
Properties
IUPAC Name |
diphenylphosphanylmethyl(diphenyl)phosphane | |
---|---|---|
Source | PubChem | |
URL | https://pubchem.ncbi.nlm.nih.gov | |
Description | Data deposited in or computed by PubChem | |
InChI |
InChI=1S/C25H22P2/c1-5-13-22(14-6-1)26(23-15-7-2-8-16-23)21-27(24-17-9-3-10-18-24)25-19-11-4-12-20-25/h1-20H,21H2 | |
Source | PubChem | |
URL | https://pubchem.ncbi.nlm.nih.gov | |
Description | Data deposited in or computed by PubChem | |
InChI Key |
XGCDBGRZEKYHNV-UHFFFAOYSA-N | |
Source | PubChem | |
URL | https://pubchem.ncbi.nlm.nih.gov | |
Description | Data deposited in or computed by PubChem | |
Canonical SMILES |
C1=CC=C(C=C1)P(CP(C2=CC=CC=C2)C3=CC=CC=C3)C4=CC=CC=C4 | |
Source | PubChem | |
URL | https://pubchem.ncbi.nlm.nih.gov | |
Description | Data deposited in or computed by PubChem | |
Molecular Formula |
C25H22P2 | |
Source | PubChem | |
URL | https://pubchem.ncbi.nlm.nih.gov | |
Description | Data deposited in or computed by PubChem | |
DSSTOX Substance ID |
DTXSID10174766 | |
Record name | Methylenebis(diphenylphosphine) | |
Source | EPA DSSTox | |
URL | https://comptox.epa.gov/dashboard/DTXSID10174766 | |
Description | DSSTox provides a high quality public chemistry resource for supporting improved predictive toxicology. | |
Molecular Weight |
384.4 g/mol | |
Source | PubChem | |
URL | https://pubchem.ncbi.nlm.nih.gov | |
Description | Data deposited in or computed by PubChem | |
Physical Description |
White crystalline powder; [Alfa Aesar MSDS] | |
Record name | Methylenebis(diphenylphosphine) | |
Source | Haz-Map, Information on Hazardous Chemicals and Occupational Diseases | |
URL | https://haz-map.com/Agents/15600 | |
Description | Haz-Map® is an occupational health database designed for health and safety professionals and for consumers seeking information about the adverse effects of workplace exposures to chemical and biological agents. | |
Explanation | Copyright (c) 2022 Haz-Map(R). All rights reserved. Unless otherwise indicated, all materials from Haz-Map are copyrighted by Haz-Map(R). No part of these materials, either text or image may be used for any purpose other than for personal use. Therefore, reproduction, modification, storage in a retrieval system or retransmission, in any form or by any means, electronic, mechanical or otherwise, for reasons other than personal use, is strictly prohibited without prior written permission. | |
CAS No. |
2071-20-7 | |
Record name | Bis(diphenylphosphino)methane | |
Source | CAS Common Chemistry | |
URL | https://commonchemistry.cas.org/detail?cas_rn=2071-20-7 | |
Description | CAS Common Chemistry is an open community resource for accessing chemical information. Nearly 500,000 chemical substances from CAS REGISTRY cover areas of community interest, including common and frequently regulated chemicals, and those relevant to high school and undergraduate chemistry classes. This chemical information, curated by our expert scientists, is provided in alignment with our mission as a division of the American Chemical Society. | |
Explanation | The data from CAS Common Chemistry is provided under a CC-BY-NC 4.0 license, unless otherwise stated. | |
Record name | 1,1-Bis(diphenylphosphino)methane | |
Source | ChemIDplus | |
URL | https://pubchem.ncbi.nlm.nih.gov/substance/?source=chemidplus&sourceid=0002071207 | |
Description | ChemIDplus is a free, web search system that provides access to the structure and nomenclature authority files used for the identification of chemical substances cited in National Library of Medicine (NLM) databases, including the TOXNET system. | |
Record name | Bis(diphenylphosphino)methane | |
Source | DTP/NCI | |
URL | https://dtp.cancer.gov/dtpstandard/servlet/dwindex?searchtype=NSC&outputformat=html&searchlist=147767 | |
Description | The NCI Development Therapeutics Program (DTP) provides services and resources to the academic and private-sector research communities worldwide to facilitate the discovery and development of new cancer therapeutic agents. | |
Explanation | Unless otherwise indicated, all text within NCI products is free of copyright and may be reused without our permission. Credit the National Cancer Institute as the source. | |
Record name | Methylenebis(diphenylphosphine) | |
Source | EPA DSSTox | |
URL | https://comptox.epa.gov/dashboard/DTXSID10174766 | |
Description | DSSTox provides a high quality public chemistry resource for supporting improved predictive toxicology. | |
Record name | Methylenebis[diphenylphosphine] | |
Source | European Chemicals Agency (ECHA) | |
URL | https://echa.europa.eu/substance-information/-/substanceinfo/100.016.541 | |
Description | The European Chemicals Agency (ECHA) is an agency of the European Union which is the driving force among regulatory authorities in implementing the EU's groundbreaking chemicals legislation for the benefit of human health and the environment as well as for innovation and competitiveness. | |
Explanation | Use of the information, documents and data from the ECHA website is subject to the terms and conditions of this Legal Notice, and subject to other binding limitations provided for under applicable law, the information, documents and data made available on the ECHA website may be reproduced, distributed and/or used, totally or in part, for non-commercial purposes provided that ECHA is acknowledged as the source: "Source: European Chemicals Agency, http://echa.europa.eu/". Such acknowledgement must be included in each copy of the material. ECHA permits and encourages organisations and individuals to create links to the ECHA website under the following cumulative conditions: Links can only be made to webpages that provide a link to the Legal Notice page. | |
Record name | 1,1-BIS(DIPHENYLPHOSPHINO)METHANE | |
Source | FDA Global Substance Registration System (GSRS) | |
URL | https://gsrs.ncats.nih.gov/ginas/app/beta/substances/I5O2HZR38V | |
Description | The FDA Global Substance Registration System (GSRS) enables the efficient and accurate exchange of information on what substances are in regulated products. Instead of relying on names, which vary across regulatory domains, countries, and regions, the GSRS knowledge base makes it possible for substances to be defined by standardized, scientific descriptions. | |
Explanation | Unless otherwise noted, the contents of the FDA website (www.fda.gov), both text and graphics, are not copyrighted. They are in the public domain and may be republished, reprinted and otherwise used freely by anyone without the need to obtain permission from FDA. Credit to the U.S. Food and Drug Administration as the source is appreciated but not required. | |
Retrosynthesis Analysis
AI-Powered Synthesis Planning: Our tool employs the Template_relevance Pistachio, Template_relevance Bkms_metabolic, Template_relevance Pistachio_ringbreaker, Template_relevance Reaxys, Template_relevance Reaxys_biocatalysis model, leveraging a vast database of chemical reactions to predict feasible synthetic routes.
One-Step Synthesis Focus: Specifically designed for one-step synthesis, it provides concise and direct routes for your target compounds, streamlining the synthesis process.
Accurate Predictions: Utilizing the extensive PISTACHIO, BKMS_METABOLIC, PISTACHIO_RINGBREAKER, REAXYS, REAXYS_BIOCATALYSIS database, our tool offers high-accuracy predictions, reflecting the latest in chemical research and data.
Strategy Settings
Precursor scoring | Relevance Heuristic |
---|---|
Min. plausibility | 0.01 |
Model | Template_relevance |
Template Set | Pistachio/Bkms_metabolic/Pistachio_ringbreaker/Reaxys/Reaxys_biocatalysis |
Top-N result to add to graph | 6 |
Feasible Synthetic Routes
Disclaimer and Information on In-Vitro Research Products
Please be aware that all articles and product information presented on BenchChem are intended solely for informational purposes. The products available for purchase on BenchChem are specifically designed for in-vitro studies, which are conducted outside of living organisms. In-vitro studies, derived from the Latin term "in glass," involve experiments performed in controlled laboratory settings using cells or tissues. It is important to note that these products are not categorized as medicines or drugs, and they have not received approval from the FDA for the prevention, treatment, or cure of any medical condition, ailment, or disease. We must emphasize that any form of bodily introduction of these products into humans or animals is strictly prohibited by law. It is essential to adhere to these guidelines to ensure compliance with legal and ethical standards in research and experimentation.