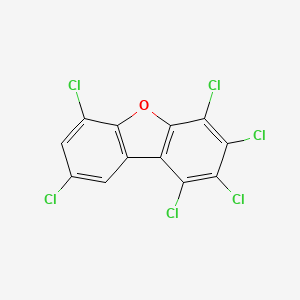
1,2,3,4,6,8-Hexachlorodibenzofuran
Overview
Description
1,2,3,4,6,8-Hexachlorodibenzofuran (CAS: 69698-60-8) is a polychlorinated dibenzofuran (PCDF) congener with six chlorine atoms substituted at positions 1, 2, 3, 4, 6, and 8 of the dibenzofuran backbone. Its molecular formula is C₁₂H₂Cl₆O, with a molecular weight of ~374.9 g/mol. It is commonly detected in environmental samples as part of complex mixtures of dioxin-like pollutants and is analyzed using gas chromatography-mass spectrometry (GC-MS) with nonane or toluene-based solutions .
Preparation Methods
General Synthetic Approaches
The synthesis of chlorinated dibenzofurans, including 1,2,3,4,6,8-Hexachlorodibenzofuran, generally involves chlorination reactions of dibenzofuran or related precursors under controlled conditions. The key challenge is selective chlorination at specific positions on the dibenzofuran ring system.
Chlorination of Dibenzofuran Precursors
- Direct chlorination of dibenzofuran or partially chlorinated dibenzofurans using chlorine gas or chlorinating agents under catalytic or thermal conditions can yield hexachlorinated congeners.
- Reaction conditions such as temperature, solvent, catalyst presence, and chlorine concentration are critical to control the degree and position of chlorination.
- For example, chlorination in the presence of catalysts like platinum on alumina (Pt/Al2O3) under oxygen atmosphere at elevated temperatures (around 360°C) has been reported for related congeners.
Oxidative Coupling and Cyclization Routes
- Some synthetic routes involve oxidative coupling of chlorinated phenols or chlorinated biphenyls followed by cyclization to form the dibenzofuran core with chlorines at desired positions.
- These methods are more complex and less commonly used for large-scale synthesis but are valuable for producing specific congeners for research.
Specific Preparation Methods for this compound
While direct literature on the exact preparation of this compound is limited, the following methods are inferred from related hexachlorodibenzofuran congeners and general chlorination chemistry:
Method | Description | Reaction Conditions | Notes |
---|---|---|---|
Catalytic Chlorination of Dibenzofuran | Dibenzofuran is chlorinated using Cl2 gas in the presence of a catalyst such as Pt/Al2O3 under oxygen atmosphere | Temperature ~360°C, pressure ~750 Torr, reaction time ~0.3 s | Produces multiple chlorinated congeners; separation required to isolate this compound |
Stepwise Chlorination of Partially Chlorinated Dibenzofurans | Starting from tetra- or pentachlorodibenzofurans, further chlorination under controlled conditions | Mild chlorination conditions to avoid over-chlorination | Allows better control of substitution pattern |
Oxidative Cyclization of Chlorinated Biphenyls or Phenols | Chlorinated biphenyls or phenols undergo oxidative cyclization to form dibenzofurans with chlorines at specific positions | Use of oxidizing agents and catalysts under reflux | More complex, used for research-scale synthesis |
Research Findings and Analytical Considerations
- Analytical methods such as high-resolution gas chromatography coupled with high-resolution mass spectrometry (HRGC/HRMS) are used to confirm the identity and purity of the compound after synthesis.
- The compound is often studied in environmental samples as a contaminant formed during industrial processes involving chlorinated compounds.
- Toxicity equivalence factors (TEFs) have been established for related hexachlorodibenzofurans, underscoring the importance of precise synthesis and analytical characterization.
Summary Table of Preparation Methods
Preparation Method | Key Reagents | Conditions | Advantages | Limitations |
---|---|---|---|---|
Catalytic Chlorination of Dibenzofuran | Dibenzofuran, Cl2, Pt/Al2O3 catalyst, O2 | 360°C, 750 Torr, short reaction time | Scalable, produces multiple congeners | Requires separation, risk of over-chlorination |
Stepwise Chlorination | Partially chlorinated dibenzofurans, Cl2 or chlorinating agents | Controlled mild conditions | Better regioselectivity | Multi-step, time-consuming |
Oxidative Cyclization | Chlorinated biphenyls/phenols, oxidants | Reflux, catalytic conditions | Specific substitution patterns achievable | Complex, low yield |
Chemical Reactions Analysis
Types of Reactions: 1,2,3,4,6,8-Hexachlorodibenzofuran undergoes various chemical reactions, including:
Oxidation: This reaction can lead to the formation of more oxidized derivatives.
Reduction: Reduction reactions can remove chlorine atoms, leading to less chlorinated dibenzofurans.
Substitution: Chlorine atoms can be substituted with other functional groups under specific conditions.
Common Reagents and Conditions:
Oxidation: Strong oxidizing agents such as potassium permanganate or ozone.
Reduction: Reducing agents like sodium borohydride or catalytic hydrogenation.
Substitution: Nucleophilic reagents such as sodium hydroxide or ammonia.
Major Products: The major products formed from these reactions depend on the specific conditions and reagents used. For example, oxidation can produce hydroxylated derivatives, while reduction can yield less chlorinated dibenzofurans .
Scientific Research Applications
Environmental Studies
Marker for Environmental Impact
1,2,3,4,6,8-Hexachlorodibenzofuran is utilized as a marker to assess the environmental impact of chlorinated compounds. Its presence in ecosystems helps researchers understand the persistence and degradation pathways of similar pollutants. Studies have shown that this compound can accumulate in sediments and biota, making it a useful indicator for environmental monitoring programs aimed at assessing contamination levels in aquatic systems.
Case Study: Sediment Analysis
A study conducted on sediment samples from the San Jacinto River revealed varying concentrations of hexachlorodibenzofurans, including this compound. The findings indicated its significant presence in the sediment matrix, which raises concerns about potential bioaccumulation in aquatic organisms .
Toxicology
Health Risk Assessment
Research on this compound has focused on its toxicological effects. It interacts with the aryl hydrocarbon receptor (AhR), leading to the activation of genes involved in xenobiotic metabolism. This mechanism is crucial for understanding the health risks associated with exposure to polychlorinated dibenzofurans .
Carcinogenic Potential
Experimental studies have indicated that exposure to this compound may increase the incidence of tumors in laboratory animals when administered alongside known carcinogens. For example, treatments involving this compound have resulted in increased rates of skin papillomas and liver tumors in mice and rats . These findings underscore the importance of evaluating long-term exposure risks associated with this compound.
Mechanism of Action
1,2,3,4,6,8-Hexachlorodibenzofuran exerts its effects primarily through interaction with the aryl hydrocarbon receptor (AhR). Upon binding to AhR, it activates the transcription of various genes involved in xenobiotic metabolism, including those encoding cytochrome P450 enzymes. This leads to the induction of phase I and phase II detoxification enzymes, which can result in both detoxification and bioactivation of the compound .
Comparison with Similar Compounds
Hexachlorodibenzofurans (HexaCDFs) comprise 135 possible congeners, differing in chlorine substitution patterns. Key isomers compared below include:
Structural and Physicochemical Properties
Table 1: Structural and Basic Physicochemical Comparisons
- Chlorination Patterns : The position of chlorine atoms critically influences molecular geometry and interactions. For example, 2,3,4,6,7,8-HexaCDF () has adjacent chlorines on both benzene rings, enhancing planarity and aryl hydrocarbon receptor (AhR) binding affinity compared to 1,2,3,4,6,8-HexaCDF .
- Lipophilicity: logP values (octanol-water partition coefficients) indicate high hydrophobicity, with 1,2,3,4,7,8-HexaCDF at 7.506, favoring bioaccumulation in fatty tissues .
Table 2: AhR Activation and Toxicity Profiles
- AhR Activation: Congeners with chlorines at 2,3,7,8 positions (e.g., 1,2,3,4,7,8-HexaCDF) exhibit stronger AhR activation, driving dioxin-like toxicity (e.g., endocrine disruption, carcinogenicity) .
Analytical and Environmental Monitoring
Biological Activity
1,2,3,4,6,8-Hexachlorodibenzofuran (HxCDF) is a member of the polychlorinated dibenzofuran (PCDF) family, known for its persistent environmental presence and potential toxicological effects. This article explores the biological activity of HxCDF, focusing on its mechanisms of action, toxicological profiles, and implications for human health and the environment.
HxCDF is characterized by its six chlorine atoms attached to a dibenzofuran structure. It acts primarily through the aryl hydrocarbon receptor (AhR) pathway. Upon binding to AhR, HxCDF translocates to the nucleus and activates transcription of genes involved in xenobiotic metabolism, notably those encoding cytochrome P450 enzymes such as CYP1A1 and CYP1B1. This activation leads to the induction of phase I and phase II detoxification enzymes, which can result in both detoxification and bioactivation processes .
Acute and Chronic Effects
Research indicates that HxCDF exhibits significant hepatotoxicity. Studies have shown that acute exposure results in liver hypertrophy and alterations in liver weight . Chronic exposure has been linked to various adverse effects including:
- Carcinogenicity : Limited evidence suggests a potential link to liver cancer in animal models . In a study involving mice treated with HxCDF after administration of carcinogens like N-methyl-N-nitro-N'-nitrosoguanidine (MNNG), an increased incidence of skin papillomas was observed .
- Teratogenicity : HxCDF has been shown to cause teratogenic effects in mice at doses not toxic to mothers. This includes developmental abnormalities and growth retardation in offspring .
- Immunotoxicity : Exposure leads to thymic atrophy and alterations in immune responses, indicating immune system disruption .
Environmental Impact
HxCDF has been utilized as a marker for assessing the environmental impact of chlorinated compounds. A study examining sediment samples from contaminated sites found varying concentrations of hexachlorodibenzofurans, including HxCDF, highlighting its persistence in ecosystems .
Human Health Studies
In epidemiological studies involving populations exposed to dioxins and related compounds, there have been correlations between exposure levels and increased rates of liver disease and cancer mortality. Notably, an assessment of a cohort exposed to HxCDF revealed a threefold increase in liver cancer mortality over 15 years .
Data Tables
Q & A
Basic Research Questions
Q. What analytical methods are recommended for quantifying 1,2,3,4,6,8-Hexachlorodibenzofuran in environmental samples?
High-resolution gas chromatography coupled with tandem mass spectrometry (HRGC/HRMS) is the gold standard for quantifying this compound at ultra-trace levels. Methodologies outlined in EPA Method 1613 and EN-1948 emphasize the use of isotopic dilution (e.g., -labeled internal standards) to correct for matrix effects and recovery losses . Detection limits typically range from 0.08–0.4 pg/L in water matrices, with strict calibration requirements (e.g., 5-point linearity checks and blank controls) to ensure precision .
Q. How does the solubility of this compound influence its environmental persistence?
Experimental solubility data for structurally similar hexachlorodibenzofurans (e.g., 1,2,3,6,7,8-HxCDF) show aqueous solubilities as low as mol/L ( g/L) at 22.5°C, contributing to bioaccumulation in lipid-rich tissues and sediments . This low solubility necessitates extraction protocols using non-polar solvents like toluene or nonane, as described in environmental analysis standards .
Q. What are the primary sources of this compound in ecosystems?
This congener is a byproduct of incomplete combustion (e.g., waste incineration) and industrial chlorination processes. Its presence in sediment cores correlates with historical industrial activity, as demonstrated in lake sediment studies where concentrations averaged pg/g in contaminated sites . Isotopic tracing (-labeled analogs) is critical for distinguishing anthropogenic vs. natural sources .
Advanced Research Questions
Q. How do isomer-specific differences in chlorination patterns affect the toxicity of this compound compared to other HxCDFs?
Toxicity varies significantly with chlorination geometry. For example, 1,2,3,4,6,7-HxCDF exhibits higher aryl hydrocarbon receptor (AhR) binding affinity in human lymphoblastoid cells than 1,2,3,7,8,9-HxCDF, with induction potencies differing by 2–3 orders of magnitude . Computational docking studies suggest lateral chlorination (positions 2,3,7,8) enhances AhR binding, while non-lateral substitutions reduce bioactivity .
Q. How can researchers resolve discrepancies in toxicity data between in vitro and in vivo models for this compound?
Discrepancies arise from metabolic dechlorination in vivo (e.g., hepatic CYP1A1/2 activity) and species-specific differences in AhR signaling pathways. For instance, 1,2,3,4,6,7-HxCDF showed potent enzyme induction in mice but lower activity in human cell lines due to differential metabolite formation . Methodological recommendations:
- Use humanized mouse models or primary hepatocyte co-cultures.
- Apply metabolomic profiling (LC-QTOF-MS) to identify active metabolites .
Q. What experimental design considerations are critical for synthesizing and purifying 1,2,3,4,6,8-HxCDF for reference standards?
Synthesis requires precise control of chlorination steps to avoid co-eluting isomers. Key steps include:
- Purification: Multi-column HPLC (e.g., PYE and C18 columns) with fraction collection guided by GC-ECD .
- Validation: Confirm purity (>99%) via HRMS and nuclear magnetic resonance (NMR) .
- Storage: Stabilize standards in 10% toluene/nonane at –20°C to prevent photodegradation .
Q. Data Contradiction Analysis
Q. Why do reported environmental concentrations of 1,2,3,4,6,8-HxCDF vary across studies?
Variability stems from:
- Matrix effects: Lipid-rich tissues (e.g., fish liver) show higher recoveries (80–120%) than soils (60–90%) due to co-extracted interferents .
- Analytical sensitivity: Older studies using GC-ECD may underreport concentrations compared to modern HRGC/HRMS .
- Geographical factors: Industrialized regions (e.g., North America) report 2–5× higher levels than remote areas .
Q. How should conflicting data on this compound’s half-life in aquatic systems be addressed?
Reported half-lives range from 2–15 years due to:
- Photolytic degradation: UV exposure in surface waters accelerates breakdown (half-life <5 years), while sediment-bound forms persist longer .
- Microbial activity: Anaerobic dechlorination by Dehalococcoides spp. reduces persistence in organic-rich sediments .
- Methodological fix: Use -labeled analogs to track degradation pathways via isotope ratio monitoring .
Properties
IUPAC Name |
1,2,3,4,6,8-hexachlorodibenzofuran | |
---|---|---|
Source | PubChem | |
URL | https://pubchem.ncbi.nlm.nih.gov | |
Description | Data deposited in or computed by PubChem | |
InChI |
InChI=1S/C12H2Cl6O/c13-3-1-4-6-7(15)8(16)9(17)10(18)12(6)19-11(4)5(14)2-3/h1-2H | |
Source | PubChem | |
URL | https://pubchem.ncbi.nlm.nih.gov | |
Description | Data deposited in or computed by PubChem | |
InChI Key |
UCFGNWHERVQWMZ-UHFFFAOYSA-N | |
Source | PubChem | |
URL | https://pubchem.ncbi.nlm.nih.gov | |
Description | Data deposited in or computed by PubChem | |
Canonical SMILES |
C1=C(C=C(C2=C1C3=C(O2)C(=C(C(=C3Cl)Cl)Cl)Cl)Cl)Cl | |
Source | PubChem | |
URL | https://pubchem.ncbi.nlm.nih.gov | |
Description | Data deposited in or computed by PubChem | |
Molecular Formula |
C12H2Cl6O | |
Source | PubChem | |
URL | https://pubchem.ncbi.nlm.nih.gov | |
Description | Data deposited in or computed by PubChem | |
DSSTOX Substance ID |
DTXSID30219986 | |
Record name | 1,2,3,4,6,8-Hexachlorodibenzofuran | |
Source | EPA DSSTox | |
URL | https://comptox.epa.gov/dashboard/DTXSID30219986 | |
Description | DSSTox provides a high quality public chemistry resource for supporting improved predictive toxicology. | |
Molecular Weight |
374.9 g/mol | |
Source | PubChem | |
URL | https://pubchem.ncbi.nlm.nih.gov | |
Description | Data deposited in or computed by PubChem | |
CAS No. |
69698-60-8 | |
Record name | 1,2,3,4,6,8-Hexachlorodibenzofuran | |
Source | ChemIDplus | |
URL | https://pubchem.ncbi.nlm.nih.gov/substance/?source=chemidplus&sourceid=0069698608 | |
Description | ChemIDplus is a free, web search system that provides access to the structure and nomenclature authority files used for the identification of chemical substances cited in National Library of Medicine (NLM) databases, including the TOXNET system. | |
Record name | 1,2,3,4,6,8-Hexachlorodibenzofuran | |
Source | EPA DSSTox | |
URL | https://comptox.epa.gov/dashboard/DTXSID30219986 | |
Description | DSSTox provides a high quality public chemistry resource for supporting improved predictive toxicology. | |
Record name | 1,2,3,4,6,8-HEXACHLORODIBENZOFURAN | |
Source | FDA Global Substance Registration System (GSRS) | |
URL | https://gsrs.ncats.nih.gov/ginas/app/beta/substances/I9A11M9ARZ | |
Description | The FDA Global Substance Registration System (GSRS) enables the efficient and accurate exchange of information on what substances are in regulated products. Instead of relying on names, which vary across regulatory domains, countries, and regions, the GSRS knowledge base makes it possible for substances to be defined by standardized, scientific descriptions. | |
Explanation | Unless otherwise noted, the contents of the FDA website (www.fda.gov), both text and graphics, are not copyrighted. They are in the public domain and may be republished, reprinted and otherwise used freely by anyone without the need to obtain permission from FDA. Credit to the U.S. Food and Drug Administration as the source is appreciated but not required. | |
Retrosynthesis Analysis
AI-Powered Synthesis Planning: Our tool employs the Template_relevance Pistachio, Template_relevance Bkms_metabolic, Template_relevance Pistachio_ringbreaker, Template_relevance Reaxys, Template_relevance Reaxys_biocatalysis model, leveraging a vast database of chemical reactions to predict feasible synthetic routes.
One-Step Synthesis Focus: Specifically designed for one-step synthesis, it provides concise and direct routes for your target compounds, streamlining the synthesis process.
Accurate Predictions: Utilizing the extensive PISTACHIO, BKMS_METABOLIC, PISTACHIO_RINGBREAKER, REAXYS, REAXYS_BIOCATALYSIS database, our tool offers high-accuracy predictions, reflecting the latest in chemical research and data.
Strategy Settings
Precursor scoring | Relevance Heuristic |
---|---|
Min. plausibility | 0.01 |
Model | Template_relevance |
Template Set | Pistachio/Bkms_metabolic/Pistachio_ringbreaker/Reaxys/Reaxys_biocatalysis |
Top-N result to add to graph | 6 |
Feasible Synthetic Routes
Disclaimer and Information on In-Vitro Research Products
Please be aware that all articles and product information presented on BenchChem are intended solely for informational purposes. The products available for purchase on BenchChem are specifically designed for in-vitro studies, which are conducted outside of living organisms. In-vitro studies, derived from the Latin term "in glass," involve experiments performed in controlled laboratory settings using cells or tissues. It is important to note that these products are not categorized as medicines or drugs, and they have not received approval from the FDA for the prevention, treatment, or cure of any medical condition, ailment, or disease. We must emphasize that any form of bodily introduction of these products into humans or animals is strictly prohibited by law. It is essential to adhere to these guidelines to ensure compliance with legal and ethical standards in research and experimentation.