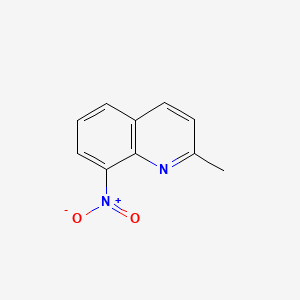
2-Methyl-8-nitroquinoline
Overview
Description
1.1. Chemical Structure and Properties 2-Methyl-8-nitroquinoline (CAS 881-07-2) is a nitro-substituted quinoline derivative with the molecular formula C₁₀H₈N₂O₂ and a molecular weight of 188.186 g/mol . The compound features a methyl group at the 2-position and a nitro group at the 8-position of the quinoline ring. Key physical properties include:
- Melting Point: 138–140°C
- Solubility: Soluble in organic solvents (e.g., DCM, THF, DMSO) but poorly soluble in water .
- Hazards: Classified as harmful (Xn) with risks of skin/eye irritation (R68) .
1.2. Synthesis The compound is synthesized via nitration of 2-methylquinoline, followed by separation of isomers (8-nitro and 5-nitro derivatives) . Oxidation of the methyl group can yield 8-nitroquinoline-2-carboxylic acid, a precursor for further functionalization .
Preparation Methods
Preparation via o-Nitrophenol and Crotonaldehyde Cyclization
This method involves the condensation of o-nitrophenol with crotonaldehyde, followed by oxidation and cyclization to form 2-methyl-8-nitroquinoline or its hydroxyquinoline precursor.
Step 1: Formation of 2-methyl-8-hydroxyquinoline
o-Nitrophenol is reduced chemically (e.g., Na2S) or catalytically (Pd/C with H2) to o-aminophenol. This intermediate then undergoes addition and dehydration with crotonaldehyde to form 2-methyl-8-hydroxyquinoline.
Step 2: Oxidation and Cyclization
The reaction mixture includes oxidants such as o-nitrophenol itself, cerium ammonium nitrate, vanadic acid, or iron oxide to facilitate dehydrogenation and ring closure.
Step 3: Conversion to this compound
The hydroxyquinoline intermediate can be further nitrated or converted to the nitro derivative under controlled conditions.
-
- Temperature: 300–320 °C
- Pressure: 1.8–2.2 MPa
- Reaction time: 4–6 hours
- Catalysts: ammonium chloride-cobalt chloride, palladium on alumina, or alumina-silica mixtures
- Solvent: ammonia water (22–28%), toluene extraction post-reaction
-
The process yields 2-methyl-8-aminoquinoline with ≥70% yield after distillation, indicating efficient conversion from the nitro precursor stage.
Preparation via Halogenated Quinoline Intermediates
An alternative route involves synthesizing 2-methyl-8-bromoquinoline by ring closure of o-bromoaniline and crotonaldehyde in the presence of oxidants and moderators, followed by amination or nitration.
-
Nitrobenzene, 2-nitrobromobenzene, cerium ammonium nitrate, vanadic acid, iron oxide.
-
Copper acetylacetonate, iron acetylacetonate, cobalt acetylacetonate, zinc acetylacetonate.
-
Dimethyl sulfoxide, N,N-dimethylformamide, acetylacetone, tetrahydrofuran, N-methylpyrrolidone.
-
Cesium carbonate, cesium hydroxide, potassium carbonate, potassium hydroxide.
-
Temperature range: 60–120 °C
This method is more complex due to difficulties in amino substitution on 2-methyl-8-chloroquinoline, but it provides an alternative pathway to nitroquinoline derivatives.
Parameter | Method 1: o-Nitrophenol Route | Method 2: Halogenated Quinoline Route |
---|---|---|
Starting Material | o-Nitrophenol | o-Bromoaniline |
Key Intermediate | 2-Methyl-8-hydroxyquinoline | 2-Methyl-8-bromoquinoline |
Oxidants | o-Nitrophenol, cerium ammonium nitrate, vanadic acid | Nitrobenzene, 2-nitrobromobenzene, cerium ammonium nitrate |
Catalysts | Ammonium chloride-cobalt chloride, Pd/Al2O3 | Copper, iron, cobalt, zinc acetylacetonates |
Solvents | Ammonia water, toluene | DMSO, DMF, acetylacetone, THF, NMP |
Temperature | 300–320 °C | 60–120 °C |
Pressure | 1.8–2.2 MPa | Atmospheric to moderate pressure |
Reaction Time | 4–6 hours | Variable, typically hours |
Yield of Final Product | ≥70% (for aminoquinoline after reduction) | Variable, dependent on substitution efficiency |
Challenges | High temperature and pressure required | Difficult amino substitution on chloroquinoline |
The use of o-nitrophenol as both oxidant and starting material allows a redox cycle where o-nitrophenol is reduced to o-aminophenol, which then reacts further, improving overall yield and efficiency.
Catalytic hydrogenation or chemical reduction (e.g., Na2S) is effective for converting nitro groups to amino groups, facilitating subsequent transformations.
The Dobner-Miller reaction catalyzed by phosphotungstic acid has been reported for related hydroxyquinoline synthesis, indicating potential alternative catalytic systems.
Amino substitution on halogenated quinolines is challenging, often limiting the practicality of halogenated intermediate routes for direct preparation of 2-methyl-8-aminoquinoline.
The preparation of this compound is best achieved via cyclization of o-nitrophenol derivatives with crotonaldehyde under oxidative conditions, followed by reduction and substitution steps. This method offers higher yields and more straightforward processing compared to halogenated intermediate routes. The use of specific oxidants and catalysts, along with controlled high-temperature and pressure conditions, is critical for optimizing the synthesis.
Chemical Reactions Analysis
Types of Reactions: 2-Methyl-8-nitroquinoline undergoes various chemical reactions, including:
Oxidation: The methyl group can be oxidized to form carboxylic acids.
Reduction: The nitro group can be reduced to an amino group.
Substitution: Electrophilic and nucleophilic substitution reactions can occur on the quinoline ring.
Common Reagents and Conditions:
Oxidation: Bromination followed by hydrolysis in aqueous sulfuric acid.
Reduction: Catalytic hydrogenation or using reducing agents like tin(II) chloride.
Substitution: Various electrophiles and nucleophiles under acidic or basic conditions.
Major Products:
Oxidation: 8-nitroquinoline-2-carboxylic acid.
Reduction: 2-methyl-8-aminoquinoline.
Substitution: Various substituted quinoline derivatives depending on the reagents used.
Scientific Research Applications
Pharmaceutical Development
Key Role in Drug Synthesis
2-Methyl-8-nitroquinoline serves as an important intermediate in the synthesis of several pharmaceutical agents, particularly anti-cancer drugs. Its unique chemical structure allows for modifications that enhance therapeutic efficacy against various cancers. For instance, it has been studied for its potential as an insulin inhibitor, showcasing its relevance in diabetes management and cancer therapy .
Case Study: Anti-Cancer Properties
Recent molecular docking studies have demonstrated that this compound exhibits promising interactions with cancer-related proteins, indicating its potential as a lead compound in drug discovery .
Study Focus | Findings |
---|---|
Molecular Docking | Inhibition of insulin-related pathways |
Synthesis of Derivatives | Enhanced cytotoxicity against cancer cell lines |
Analytical Chemistry
Fluorescent Probes
The compound is utilized as a fluorescent probe in analytical methods for detecting and quantifying biomolecules in complex mixtures. Its fluorescence properties make it suitable for applications such as high-performance liquid chromatography (HPLC) and other spectroscopic techniques.
Application Example: Biomolecule Detection
In one study, this compound was effectively employed to detect specific proteins in biological samples, demonstrating its utility in clinical diagnostics .
Analytical Method | Application |
---|---|
High-Performance Liquid Chromatography (HPLC) | Detection of proteins in serum samples |
Material Science
Advanced Materials Formulation
In material science, this compound is incorporated into the formulation of polymers and coatings. Its chemical properties enhance the durability and performance of these materials, making them suitable for industrial applications.
Case Study: Coating Applications
Research indicates that adding this compound to polymer matrices results in improved resistance to environmental degradation, which is crucial for outdoor applications .
Material Type | Performance Improvement |
---|---|
Polymers | Increased UV resistance |
Coatings | Enhanced durability against weathering |
Biochemical Research
Enzyme Inhibition Studies
Researchers utilize this compound to study enzyme inhibition and receptor binding. Its ability to interact with various biological targets provides insights into metabolic pathways and therapeutic targets.
Example: Enzyme Interaction
Studies have shown that this compound can inhibit specific enzymes involved in metabolic disorders, paving the way for new therapeutic strategies .
Research Focus | Outcome |
---|---|
Enzyme Inhibition | Identification of potential drug targets |
Environmental Monitoring
Pollutant Detection
The compound is also applied in environmental monitoring to detect pollutants in water and soil samples. Its sensitivity allows for the identification of hazardous substances, aiding compliance with environmental regulations.
Case Study: Water Quality Monitoring
In an industrial setting, this compound was used to monitor heavy metal contamination in wastewater, proving effective in ensuring regulatory compliance .
Monitoring Type | Application Example |
---|---|
Water Quality | Detection of heavy metals |
Soil Samples | Monitoring hazardous substances |
Mechanism of Action
The mechanism of action of 2-Methyl-8-nitroquinoline involves its interaction with molecular targets and pathways within biological systems. The nitro group can undergo bioreduction to form reactive intermediates that interact with cellular components, leading to various biological effects. The quinoline ring system can intercalate with DNA, inhibiting DNA synthesis and function .
Comparison with Similar Compounds
Comparison with Structural Analogs
Positional Isomers: 4-Methyl-8-nitroquinoline
Structure : Methyl group at the 4-position instead of 2 (CAS 2801-29-8; C₁₀H₈N₂O₂) .
Key Differences :
- Electronic Effects : The 4-methyl group exerts minimal steric hindrance but may alter electron density distribution compared to the 2-methyl isomer.
- Applications: Limited data, but positional differences may influence coordination chemistry in ligand design .
Functionalized Derivatives
2.2.1. 2-Methyl-8-(4-nitrophenoxy)quinoline
- Structure: Incorporates a 4-nitrophenoxy group at the 8-position (CAS 1846-92-0; C₁₆H₁₂N₂O₃) .
- Applications: Not explicitly stated, but extended conjugation may suit optoelectronic materials.
2.2.2. 3-Fluoro-8-nitroquinoline
- Structure : Fluorine at the 3-position (CAS 236093-08-6; C₉H₅FN₂O₂) .
- Reactivity : Fluorine’s electronegativity may stabilize the nitro group, affecting reduction kinetics.
- Applications: Potential use in medicinal chemistry, though specific studies are lacking.
2.2.3. 8-Amino Derivatives
- Synthesis: Photocatalytic reduction of 2-methyl-8-nitroquinoline yields 2-methylquinolin-8-amine with >90% efficiency .
- Applications : Amines serve as intermediates for pharmaceuticals (e.g., antimalarials) and ligands.
Comparative Analysis Table
Discussion of Substituent Effects
- Steric vs. Electronic Influence: The 2-methyl group introduces steric hindrance near the quinoline nitrogen, affecting coordination in metal complexes (e.g., Cu ligands in ). 4-methyl substitution minimizes steric effects but may enhance π-system delocalization .
- Nitro Group Reactivity: Reduction of the nitro group in this compound is highly efficient under photocatalytic conditions (>90% yield) , whereas derivatives with electron-withdrawing groups (e.g., nitrophenoxy) may require harsher conditions.
- Biological Activity: this compound derivatives exhibit antimalarial activity (Atokel series) , while fluorinated analogs (e.g., 3-Fluoro-8-nitroquinoline) remain unexplored in this context.
Biological Activity
2-Methyl-8-nitroquinoline (2M8NQ) is a compound belonging to the quinoline family, which has garnered attention due to its diverse biological activities. This article explores the biological activity of 2M8NQ, focusing on its anticancer properties, mechanisms of action, and potential therapeutic applications.
Chemical Structure and Properties
This compound has the molecular formula and is characterized by a nitro group at the 8-position and a methyl group at the 2-position of the quinoline ring. This specific arrangement contributes to its unique biological properties.
Anticancer Activity
Recent studies have highlighted the anticancer potential of 2M8NQ. It has been reported to exhibit significant cytotoxic effects against various cancer cell lines, including:
- Breast cancer (MCF-7)
- Lung cancer (A549)
- Colon cancer (HCT116)
The anticancer activity of 2M8NQ is believed to be mediated through several mechanisms:
- Induction of Apoptosis : 2M8NQ triggers programmed cell death in cancer cells by activating caspase pathways.
- Cell Cycle Arrest : The compound has been shown to induce G1 phase arrest in cancer cells, preventing their proliferation.
- Inhibition of Angiogenesis : It disrupts the formation of new blood vessels that tumors require for growth.
Study 1: Cytotoxicity Evaluation
In a study published in ResearchGate, 2M8NQ was evaluated for its cytotoxic effects on various cancer cell lines. The findings indicated that:
- MCF-7 Cells : IC50 = 15 µM
- A549 Cells : IC50 = 12 µM
- HCT116 Cells : IC50 = 10 µM
These results suggest that 2M8NQ exhibits potent cytotoxicity, particularly against colon cancer cells (HCT116) .
Study 2: Molecular Docking Studies
A molecular docking study conducted on 2M8NQ revealed its potential as an inhibitor of specific protein targets involved in cancer progression. The binding affinity was assessed against various targets, showing promising interactions with:
Target Protein | Binding Affinity (kcal/mol) |
---|---|
Bcl-2 | -9.5 |
VEGFR | -8.7 |
EGFR | -7.9 |
These interactions indicate that 2M8NQ may effectively inhibit pathways critical for tumor growth and survival .
Toxicological Profile
While exploring the biological activities, it is essential to consider the toxicological profile of 2M8NQ. Data from PubChem indicates that:
Q & A
Q. Basic: What are the optimal synthetic routes for preparing 2-methyl-8-nitroquinoline, and how do reaction conditions influence yield?
Answer:
The synthesis of this compound typically involves nitration of 2-methylquinoline or functionalization of pre-substituted quinoline derivatives. Key methodologies include:
- Direct nitration : Controlled nitration using mixed acid (HNO₃/H₂SO₄) at 0–5°C to avoid over-nitration. Yields depend on stoichiometry and reaction time .
- Reductive methods : For derivatives requiring nitro group reduction, NaBH₃CN or H₂/Pd systems are preferred for selectivity, as seen in analogous quinoline syntheses .
- Purity optimization : Post-synthesis purification via recrystallization (e.g., using ethanol/water) improves purity to >95%, critical for reproducibility .
Q. Basic: How can researchers confirm the identity and purity of this compound experimentally?
Answer:
- Spectroscopic analysis : Use -NMR to verify the methyl group (δ ~2.6 ppm) and nitro group substitution patterns. IR spectroscopy confirms nitro stretches (~1520 cm⁻¹ and ~1350 cm⁻¹) .
- Chromatography : HPLC or GC-MS (retention time comparison with standards) ensures purity >95% .
- Melting point : A sharp mp of 138°C (lit. value) confirms crystallinity and absence of impurities .
Q. Basic: What safety protocols are critical when handling this compound in the lab?
Answer:
- PPE : Wear nitrile gloves, lab coats, and safety goggles due to skin/eye irritation risks (GHS Category 2/2A) .
- Ventilation : Use fume hoods to avoid inhalation; the compound is flagged under R68 (possible mutagen) .
- Waste disposal : Neutralize nitro-containing waste with reducing agents (e.g., FeSO₄) before disposal to prevent environmental contamination .
Q. Advanced: How can the nitro group in this compound be selectively functionalized for medicinal chemistry applications?
Answer:
- Reduction to amine : Catalytic hydrogenation (H₂/Pd-C) or NaBH₃CN in acidic media converts the nitro group to an amine, enabling coupling reactions for drug analogs .
- Electrophilic substitution : The nitro group directs further substitution (e.g., halogenation) at the 5- or 7-positions of the quinoline ring, as demonstrated in antimalarial derivative syntheses .
- Photocatalytic modifications : Recent studies suggest using UV light with Ru-based catalysts for C–N bond formation, though yields require optimization .
Q. Advanced: What analytical challenges arise when distinguishing this compound from structural isomers?
Answer:
- X-ray crystallography : Resolves positional isomerism (e.g., 3-nitro vs. 8-nitro) via unit cell parameters and molecular packing analysis .
- Mass spectrometry : High-resolution MS (HRMS) differentiates isomers by exact mass and fragmentation patterns (e.g., loss of NO₂ versus CH₃ groups) .
- Computational modeling : DFT calculations predict -NMR chemical shifts to validate experimental data .
Q. Advanced: How should researchers address contradictory data in the literature regarding this compound’s reactivity?
Answer:
- Systematic review : Follow PRISMA guidelines to extract and compare data from primary sources, focusing on variables like solvent polarity, temperature, and catalyst loadings .
- Controlled replication : Reproduce key studies under standardized conditions (e.g., anhydrous solvents, inert atmosphere) to isolate confounding factors .
- Statistical analysis : Apply ANOVA or t-tests to evaluate significance of discrepancies in reported reaction yields or spectroscopic data .
Q. Advanced: What strategies ensure robust data reporting for this compound in publications?
Answer:
- Detailed methods : Include exact molar ratios, purification gradients, and instrument calibration details (e.g., NMR spectrometer frequency) to enable replication .
- Supplementary data : Provide raw spectral files, crystallographic data (CIF), and HPLC chromatograms in supporting information .
- Error analysis : Quantify uncertainties (e.g., ±0.5°C in mp measurements) and discuss limitations of analytical techniques .
Q. Advanced: How can researchers interpret ambiguous spectroscopic data for this compound derivatives?
Answer:
- 2D-NMR : Use COSY and NOESY to resolve overlapping proton signals in complex mixtures .
- Isotopic labeling : Synthesize - or -labeled analogs to track nitro group behavior in IR/Raman spectra .
- Comparative databases : Cross-reference with NIST Chemistry WebBook or ECHA databases for spectral validation .
Q. Advanced: What methodologies are recommended for studying this compound’s environmental toxicity?
Answer:
- OECD guidelines : Perform Daphnia magna acute toxicity tests (EC₅₀) and algal growth inhibition assays to assess ecotoxicity .
- Degradation studies : Use LC-MS to track photolytic or microbial degradation products in simulated wastewater .
- QSAR modeling : Predict bioaccumulation potential using logP values (calculated: ~2.1) and molecular descriptors .
Properties
IUPAC Name |
2-methyl-8-nitroquinoline | |
---|---|---|
Source | PubChem | |
URL | https://pubchem.ncbi.nlm.nih.gov | |
Description | Data deposited in or computed by PubChem | |
InChI |
InChI=1S/C10H8N2O2/c1-7-5-6-8-3-2-4-9(12(13)14)10(8)11-7/h2-6H,1H3 | |
Source | PubChem | |
URL | https://pubchem.ncbi.nlm.nih.gov | |
Description | Data deposited in or computed by PubChem | |
InChI Key |
UHPGVDHXHDPYQP-UHFFFAOYSA-N | |
Source | PubChem | |
URL | https://pubchem.ncbi.nlm.nih.gov | |
Description | Data deposited in or computed by PubChem | |
Canonical SMILES |
CC1=NC2=C(C=CC=C2[N+](=O)[O-])C=C1 | |
Source | PubChem | |
URL | https://pubchem.ncbi.nlm.nih.gov | |
Description | Data deposited in or computed by PubChem | |
Molecular Formula |
C10H8N2O2 | |
Source | PubChem | |
URL | https://pubchem.ncbi.nlm.nih.gov | |
Description | Data deposited in or computed by PubChem | |
DSSTOX Substance ID |
DTXSID30236815 | |
Record name | Quinaldine, 8-nitro- | |
Source | EPA DSSTox | |
URL | https://comptox.epa.gov/dashboard/DTXSID30236815 | |
Description | DSSTox provides a high quality public chemistry resource for supporting improved predictive toxicology. | |
Molecular Weight |
188.18 g/mol | |
Source | PubChem | |
URL | https://pubchem.ncbi.nlm.nih.gov | |
Description | Data deposited in or computed by PubChem | |
CAS No. |
881-07-2 | |
Record name | 2-Methyl-8-nitroquinoline | |
Source | CAS Common Chemistry | |
URL | https://commonchemistry.cas.org/detail?cas_rn=881-07-2 | |
Description | CAS Common Chemistry is an open community resource for accessing chemical information. Nearly 500,000 chemical substances from CAS REGISTRY cover areas of community interest, including common and frequently regulated chemicals, and those relevant to high school and undergraduate chemistry classes. This chemical information, curated by our expert scientists, is provided in alignment with our mission as a division of the American Chemical Society. | |
Explanation | The data from CAS Common Chemistry is provided under a CC-BY-NC 4.0 license, unless otherwise stated. | |
Record name | 2-Methyl-8-nitroquinoline | |
Source | ChemIDplus | |
URL | https://pubchem.ncbi.nlm.nih.gov/substance/?source=chemidplus&sourceid=0000881072 | |
Description | ChemIDplus is a free, web search system that provides access to the structure and nomenclature authority files used for the identification of chemical substances cited in National Library of Medicine (NLM) databases, including the TOXNET system. | |
Record name | QUINALDINE, 8-NITRO- | |
Source | DTP/NCI | |
URL | https://dtp.cancer.gov/dtpstandard/servlet/dwindex?searchtype=NSC&outputformat=html&searchlist=163380 | |
Description | The NCI Development Therapeutics Program (DTP) provides services and resources to the academic and private-sector research communities worldwide to facilitate the discovery and development of new cancer therapeutic agents. | |
Explanation | Unless otherwise indicated, all text within NCI products is free of copyright and may be reused without our permission. Credit the National Cancer Institute as the source. | |
Record name | Quinaldine, 8-nitro- | |
Source | EPA DSSTox | |
URL | https://comptox.epa.gov/dashboard/DTXSID30236815 | |
Description | DSSTox provides a high quality public chemistry resource for supporting improved predictive toxicology. | |
Record name | 2-methyl-8-nitroquinoline | |
Source | European Chemicals Agency (ECHA) | |
URL | https://echa.europa.eu/substance-information/-/substanceinfo/100.011.745 | |
Description | The European Chemicals Agency (ECHA) is an agency of the European Union which is the driving force among regulatory authorities in implementing the EU's groundbreaking chemicals legislation for the benefit of human health and the environment as well as for innovation and competitiveness. | |
Explanation | Use of the information, documents and data from the ECHA website is subject to the terms and conditions of this Legal Notice, and subject to other binding limitations provided for under applicable law, the information, documents and data made available on the ECHA website may be reproduced, distributed and/or used, totally or in part, for non-commercial purposes provided that ECHA is acknowledged as the source: "Source: European Chemicals Agency, http://echa.europa.eu/". Such acknowledgement must be included in each copy of the material. ECHA permits and encourages organisations and individuals to create links to the ECHA website under the following cumulative conditions: Links can only be made to webpages that provide a link to the Legal Notice page. | |
Record name | 2-METHYL-8-NITROQUINOLINE | |
Source | FDA Global Substance Registration System (GSRS) | |
URL | https://gsrs.ncats.nih.gov/ginas/app/beta/substances/XRV949AUQZ | |
Description | The FDA Global Substance Registration System (GSRS) enables the efficient and accurate exchange of information on what substances are in regulated products. Instead of relying on names, which vary across regulatory domains, countries, and regions, the GSRS knowledge base makes it possible for substances to be defined by standardized, scientific descriptions. | |
Explanation | Unless otherwise noted, the contents of the FDA website (www.fda.gov), both text and graphics, are not copyrighted. They are in the public domain and may be republished, reprinted and otherwise used freely by anyone without the need to obtain permission from FDA. Credit to the U.S. Food and Drug Administration as the source is appreciated but not required. | |
Retrosynthesis Analysis
AI-Powered Synthesis Planning: Our tool employs the Template_relevance Pistachio, Template_relevance Bkms_metabolic, Template_relevance Pistachio_ringbreaker, Template_relevance Reaxys, Template_relevance Reaxys_biocatalysis model, leveraging a vast database of chemical reactions to predict feasible synthetic routes.
One-Step Synthesis Focus: Specifically designed for one-step synthesis, it provides concise and direct routes for your target compounds, streamlining the synthesis process.
Accurate Predictions: Utilizing the extensive PISTACHIO, BKMS_METABOLIC, PISTACHIO_RINGBREAKER, REAXYS, REAXYS_BIOCATALYSIS database, our tool offers high-accuracy predictions, reflecting the latest in chemical research and data.
Strategy Settings
Precursor scoring | Relevance Heuristic |
---|---|
Min. plausibility | 0.01 |
Model | Template_relevance |
Template Set | Pistachio/Bkms_metabolic/Pistachio_ringbreaker/Reaxys/Reaxys_biocatalysis |
Top-N result to add to graph | 6 |
Feasible Synthetic Routes
Disclaimer and Information on In-Vitro Research Products
Please be aware that all articles and product information presented on BenchChem are intended solely for informational purposes. The products available for purchase on BenchChem are specifically designed for in-vitro studies, which are conducted outside of living organisms. In-vitro studies, derived from the Latin term "in glass," involve experiments performed in controlled laboratory settings using cells or tissues. It is important to note that these products are not categorized as medicines or drugs, and they have not received approval from the FDA for the prevention, treatment, or cure of any medical condition, ailment, or disease. We must emphasize that any form of bodily introduction of these products into humans or animals is strictly prohibited by law. It is essential to adhere to these guidelines to ensure compliance with legal and ethical standards in research and experimentation.