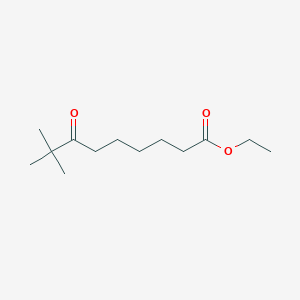
Ethyl 8,8-dimethyl-7-oxononanoate
Overview
Description
Ethyl 8,8-dimethyl-7-oxononanoate (C₁₂H₂₂O₃) is a branched aliphatic ester featuring a ketone group at the 7th carbon and two methyl substituents at the 8th carbon. This structural configuration distinguishes it from linear or aromatic-substituted esters.
Preparation Methods
Synthetic Routes and Reaction Conditions: Ethyl 8,8-dimethyl-7-oxononanoate can be synthesized through several methods. One common approach involves the esterification of 8,8-dimethyl-7-oxononanoic acid with ethanol in the presence of a strong acid catalyst such as sulfuric acid. The reaction is typically carried out under reflux conditions to ensure complete conversion.
Industrial Production Methods: In an industrial setting, the production of this compound may involve continuous flow processes to enhance efficiency and yield. The use of automated systems and optimized reaction conditions, such as controlled temperature and pressure, can further improve the scalability of the synthesis.
Chemical Reactions Analysis
Types of Reactions: Ethyl 8,8-dimethyl-7-oxononanoate undergoes various chemical reactions, including:
Oxidation: The ketone group can be oxidized to form a carboxylic acid.
Reduction: The ketone group can be reduced to form an alcohol.
Substitution: The ester group can undergo nucleophilic substitution reactions.
Common Reagents and Conditions:
Oxidation: Reagents such as potassium permanganate or chromium trioxide can be used under acidic conditions.
Reduction: Reagents such as sodium borohydride or lithium aluminum hydride can be used under anhydrous conditions.
Substitution: Nucleophiles such as amines or alcohols can be used in the presence of a base like sodium hydroxide.
Major Products:
Oxidation: 8,8-dimethyl-7-oxononanoic acid.
Reduction: 8,8-dimethyl-7-hydroxynonanoate.
Substitution: Various substituted esters depending on the nucleophile used.
Scientific Research Applications
Applications in Organic Synthesis
- Intermediate in Synthesis : Ethyl 8,8-dimethyl-7-oxononanoate serves as a valuable intermediate in the synthesis of more complex organic molecules. Its unique structural features enhance its reactivity, making it suitable for various chemical transformations such as oxidation and reduction.
- Building Block for Derivatives : This compound can be utilized to generate derivatives that possess different properties and functionalities. For instance, it can be converted into other esters or ketones through selective reactions.
- Green Chemistry : The compound's synthesis can be adapted for greener synthetic pathways, which are increasingly important in modern chemistry. For example, utilizing less hazardous reagents and optimizing reaction conditions can minimize environmental impact .
Case Study 1: Synthesis of Sophorolipid Aldehyde
A study demonstrated the use of this compound as a precursor for synthesizing peracetylated sophorolipid aldehyde. The compound was subjected to ozonolysis followed by reductive work-up, showcasing its utility in synthesizing bioactive lipids .
Case Study 2: Antiproliferative Activity
Research explored the antiproliferative activity of compounds derived from this compound. These derivatives exhibited significant activity against various cancer cell lines, highlighting their potential in pharmaceutical applications .
Mechanism of Action
The mechanism of action of ethyl 8,8-dimethyl-7-oxononanoate depends on the specific reactions it undergoes. For example, in reduction reactions, the ketone group is reduced to an alcohol through the transfer of hydride ions. The molecular targets and pathways involved vary based on the specific application and reaction conditions.
Comparison with Similar Compounds
Comparison with Structurally Similar Compounds
Ethyl 7-oxononanoate (C₁₁H₂₀O₃)
Structural Differences :
- Linear aliphatic chain with a ketone at position 7.
- Lacks methyl branching at position 8.
Physical Properties :
- Molecular weight: 200.27 g/mol (vs. 214.31 g/mol for the dimethyl analog).
- Higher solubility in polar solvents due to reduced steric hindrance.
- Lower boiling point compared to branched analogs due to weaker intermolecular forces .
Aromatic-Substituted Esters (e.g., Ethyl 8-(3,4-dimethoxyphenyl)-8-oxooctanoate, C₁₈H₂₆O₅)
Structural Differences :
- Aromatic substituents (e.g., dimethoxyphenyl) at the ketone-bearing carbon.
- Extended carbon chains compared to aliphatic analogs.
Physical Properties :
- Higher molecular weight (322.4 g/mol) due to aromatic groups.
- Reduced solubility in polar solvents but enhanced stability in organic matrices .
Cyclic and Heterocyclic Esters (e.g., 8-O-Acetylshanzhiside Methyl Ester)
Structural Differences :
- Complex cyclic frameworks with multiple functional groups (e.g., acetyloxy, hydroxyl).
- No structural resemblance to linear or branched aliphatic esters.
Comparative Data Table
Key Research Findings
- Role of 7-Oxo Group: The ketone at position 7 in Ethyl 7-oxononanoate is critical in enzymatic reactions, such as those catalyzed by AONS, which is essential for biotin biosynthesis. This suggests that ethyl esters with 7-oxo groups may serve as substrates or inhibitors in related biochemical pathways .
- Impact of Branching: The dimethyl substitution in this compound likely reduces its volatility and increases steric hindrance, making it less reactive in nucleophilic reactions compared to linear analogs .
- Aromatic vs. Aliphatic : Aromatic-substituted esters exhibit enhanced stability and altered electronic properties, enabling their use in targeted drug design .
Biological Activity
Ethyl 8,8-dimethyl-7-oxononanoate (CAS No. 898776-42-6) is a compound of increasing interest due to its potential biological activities. This article explores its chemical properties, biological effects, and relevant research findings.
- Molecular Formula : C13H24O3
- Molecular Weight : 228.33 g/mol
- Synonyms : Ethyl 8,8-dimethyl-7-oxo-nonanoate; Nonanoic acid, 8,8-dimethyl-7-oxo-, ethyl ester
Antimicrobial Activity
This compound has shown promising antimicrobial properties. In a study examining various compounds for their minimum inhibitory concentration (MIC) against different bacterial strains, it was found that:
Compound | MIC (µg/mL) | Target Bacteria |
---|---|---|
This compound | 5 | Staphylococcus aureus |
10 | Bacillus subtilis | |
6 | Enterococcus faecium | |
5 | Streptococcus pneumoniae |
These results indicate that the compound exhibits significant antibacterial activity against both Gram-positive and Gram-negative bacteria .
Anti-inflammatory Effects
Research has also indicated that this compound may possess anti-inflammatory properties. In vitro studies demonstrated that the compound inhibited the expression of pro-inflammatory cytokines such as IL-1β and TNF-α in lipopolysaccharide-stimulated RAW264.7 macrophages at concentrations as low as 10 µM . This suggests a potential role for the compound in modulating inflammatory responses.
The biological activity of this compound can be attributed to its structural characteristics, which allow it to interact with various biological pathways:
- Enzyme Inhibition : The compound's structure may facilitate inhibition of key enzymes involved in bacterial cell wall synthesis and inflammatory pathways.
- Free Radical Scavenging : Similar compounds have been noted for their ability to scavenge free radicals, contributing to their antimicrobial and anti-inflammatory effects .
Study on Antibacterial Activity
In a comparative study of various fatty acid derivatives, this compound was highlighted for its low MIC values against several pathogenic bacteria. The study concluded that modifications to the alkyl side chain significantly enhanced the compound's bioactivity .
Study on Anti-inflammatory Properties
A separate investigation into the anti-inflammatory effects of similar compounds revealed that this compound could effectively reduce inflammation markers in vitro. The findings suggested that its application could be beneficial in therapeutic contexts where inflammation plays a critical role .
Q & A
Basic Research Questions
Q. What experimental methodologies are recommended for synthesizing Ethyl 8,8-dimethyl-7-oxononanoate in laboratory settings?
Synthesis of this compound requires careful selection of precursors (e.g., pimeloyl-CoA analogs or branched-chain keto acids) and catalysts (e.g., pyridoxal 5'-phosphate-dependent enzymes). Key steps include:
- Condensation reactions : Use decarboxylative condensation protocols similar to those in biotin biosynthesis, where AONS catalyzes l-alanine and pimeloyl-CoA .
- Stereochemical control : Optimize reaction conditions (pH, temperature) to ensure stereospecificity, as seen in AONS-catalyzed formation of 8(S)-amino-7-oxononanoate .
- Purification : Employ column chromatography or HPLC to isolate the esterified product, ensuring purity via NMR or mass spectrometry .
Q. How can spectroscopic techniques validate the structural integrity of this compound?
- NMR spectroscopy : Use H and C NMR to confirm methyl branching (8,8-dimethyl) and ketone (7-oxo) positions. Compare chemical shifts with analogous compounds like 8-amino-7-oxononanoate .
- Mass spectrometry (MS) : High-resolution MS (HRMS) ensures molecular formula accuracy (e.g., [M+H] for CHO).
- Infrared (IR) spectroscopy : Identify carbonyl (C=O) stretches (~1700 cm) and ester (C-O) vibrations (~1250 cm) .
Q. What biochemical pathways or enzymes might interact with this compound?
The compound’s structural similarity to 7-oxononanoate derivatives suggests potential roles in:
- Biotin biosynthesis : Investigate interactions with AONS homologs, which bind pimeloyl-CoA and catalyze decarboxylative condensations .
- Fatty acid metabolism : Screen for activity with acyl-CoA synthetases or thioesterases due to its ester and ketone functional groups .
Advanced Research Questions
Q. How can X-ray crystallography resolve conformational dynamics of this compound during enzymatic reactions?
- Crystal structure determination : Co-crystallize the compound with target enzymes (e.g., AONS homologs) to map binding pockets. Use synchrotron radiation for high-resolution (<2.0 Å) data .
- Active site analysis : Identify residues (e.g., Lys236 in AONS) critical for substrate orientation and catalysis through mutagenesis and structural comparisons .
- Time-resolved crystallography : Capture intermediate states (e.g., external aldimine or quinonoid) to elucidate reaction mechanisms .
Q. What computational approaches predict the reactivity of this compound in catalytic systems?
- Density Functional Theory (DFT) : Calculate transition states and energy barriers for decarboxylation or ester hydrolysis. Include solvent effects via Polarizable Continuum Models (PCM) .
- Molecular Dynamics (MD) : Simulate enzyme-substrate interactions to assess binding affinity and conformational changes (e.g., C-terminal domain motion in AONS) .
- Docking studies : Use software like AutoDock to screen for potential enzyme targets based on steric and electronic complementarity .
Q. How can kinetic isotope effects (KIEs) and transient kinetics elucidate the catalytic mechanism of this compound in enzyme assays?
- Deuterium labeling : Introduce H at the α-carbon of the ester group to measure primary KIEs, indicating rate-limiting steps (e.g., proton abstraction) .
- Stopped-flow spectroscopy : Monitor quinonoid intermediate formation (λ = 500–550 nm) upon mixing the compound with enzymes and cofactors .
- Pre-steady-state analysis : Fit kinetic data to models (e.g., Michaelis-Menten with burst phases) to distinguish binding vs. catalytic steps .
Q. What strategies address contradictory data in characterizing this compound's thermodynamic properties?
- Calorimetric validation : Compare experimental enthalpy (ΔH) and entropy (ΔS) values from ITC with computational predictions .
- Error analysis : Quantify uncertainties in spectroscopic or chromatographic data using statistical tools (e.g., standard deviation of triplicate runs) .
- Cross-method verification : Correlate results from DSC (thermal stability) and DFT (bond dissociation energies) to resolve discrepancies .
Properties
IUPAC Name |
ethyl 8,8-dimethyl-7-oxononanoate | |
---|---|---|
Source | PubChem | |
URL | https://pubchem.ncbi.nlm.nih.gov | |
Description | Data deposited in or computed by PubChem | |
InChI |
InChI=1S/C13H24O3/c1-5-16-12(15)10-8-6-7-9-11(14)13(2,3)4/h5-10H2,1-4H3 | |
Source | PubChem | |
URL | https://pubchem.ncbi.nlm.nih.gov | |
Description | Data deposited in or computed by PubChem | |
InChI Key |
WNABFIITZQAWAC-UHFFFAOYSA-N | |
Source | PubChem | |
URL | https://pubchem.ncbi.nlm.nih.gov | |
Description | Data deposited in or computed by PubChem | |
Canonical SMILES |
CCOC(=O)CCCCCC(=O)C(C)(C)C | |
Source | PubChem | |
URL | https://pubchem.ncbi.nlm.nih.gov | |
Description | Data deposited in or computed by PubChem | |
Molecular Formula |
C13H24O3 | |
Source | PubChem | |
URL | https://pubchem.ncbi.nlm.nih.gov | |
Description | Data deposited in or computed by PubChem | |
DSSTOX Substance ID |
DTXSID30645665 | |
Record name | Ethyl 8,8-dimethyl-7-oxononanoate | |
Source | EPA DSSTox | |
URL | https://comptox.epa.gov/dashboard/DTXSID30645665 | |
Description | DSSTox provides a high quality public chemistry resource for supporting improved predictive toxicology. | |
Molecular Weight |
228.33 g/mol | |
Source | PubChem | |
URL | https://pubchem.ncbi.nlm.nih.gov | |
Description | Data deposited in or computed by PubChem | |
CAS No. |
898776-42-6 | |
Record name | Ethyl 8,8-dimethyl-7-oxononanoate | |
Source | EPA DSSTox | |
URL | https://comptox.epa.gov/dashboard/DTXSID30645665 | |
Description | DSSTox provides a high quality public chemistry resource for supporting improved predictive toxicology. | |
Disclaimer and Information on In-Vitro Research Products
Please be aware that all articles and product information presented on BenchChem are intended solely for informational purposes. The products available for purchase on BenchChem are specifically designed for in-vitro studies, which are conducted outside of living organisms. In-vitro studies, derived from the Latin term "in glass," involve experiments performed in controlled laboratory settings using cells or tissues. It is important to note that these products are not categorized as medicines or drugs, and they have not received approval from the FDA for the prevention, treatment, or cure of any medical condition, ailment, or disease. We must emphasize that any form of bodily introduction of these products into humans or animals is strictly prohibited by law. It is essential to adhere to these guidelines to ensure compliance with legal and ethical standards in research and experimentation.