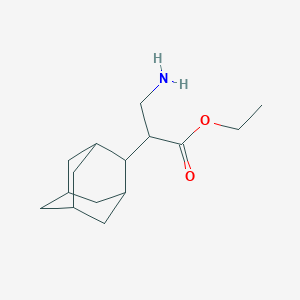
Ethyl 2-(adamantan-2-yl)-3-aminopropanoate
- Click on QUICK INQUIRY to receive a quote from our team of experts.
- With the quality product at a COMPETITIVE price, you can focus more on your research.
Overview
Description
Ethyl 2-(adamantan-2-yl)-3-aminopropanoate is a compound that features an adamantane core structure, which is known for its stability and unique three-dimensional framework
Preparation Methods
Synthetic Routes and Reaction Conditions
The synthesis of ethyl 2-(adamantan-2-yl)-3-aminopropanoate typically involves the reaction of adamantan-2-yl derivatives with ethyl 3-aminopropanoate under specific conditions. One common method includes the use of a base such as triethylamine in an organic solvent like diethyl ether at room temperature . The reaction proceeds through nucleophilic substitution, where the amino group of the ethyl 3-aminopropanoate attacks the adamantane derivative, forming the desired product.
Industrial Production Methods
Industrial production of this compound may involve similar synthetic routes but on a larger scale. The process would be optimized for yield and purity, often involving continuous flow reactors and automated systems to ensure consistent production. The use of catalysts and optimized reaction conditions can further enhance the efficiency of the synthesis.
Chemical Reactions Analysis
Types of Reactions
Ethyl 2-(adamantan-2-yl)-3-aminopropanoate can undergo various chemical reactions, including:
Oxidation: The compound can be oxidized to form corresponding oxides or ketones.
Reduction: Reduction reactions can convert the ester group to an alcohol.
Substitution: The amino group can participate in substitution reactions, forming new derivatives.
Common Reagents and Conditions
Oxidation: Common oxidizing agents include potassium permanganate (KMnO4) and chromium trioxide (CrO3).
Reduction: Reducing agents such as lithium aluminum hydride (LiAlH4) or sodium borohydride (NaBH4) are typically used.
Substitution: Reagents like alkyl halides or acyl chlorides can be used in substitution reactions, often in the presence of a base.
Major Products Formed
The major products formed from these reactions depend on the specific conditions and reagents used. For example, oxidation may yield adamantanone derivatives, while reduction can produce alcohols. Substitution reactions can lead to a variety of new compounds with different functional groups attached to the adamantane core.
Scientific Research Applications
Ethyl 2-(adamantan-2-yl)-3-aminopropanoate has several scientific research applications:
Chemistry: It is used as a building block for synthesizing more complex molecules and studying reaction mechanisms.
Biology: The compound can be used in the development of bioactive molecules, including potential pharmaceuticals.
Medicine: Research into its potential as a drug candidate for treating various diseases is ongoing, particularly due to the stability and unique properties of the adamantane core.
Industry: It finds applications in the development of new materials, including polymers and nanomaterials, due to its structural properties.
Mechanism of Action
The mechanism by which ethyl 2-(adamantan-2-yl)-3-aminopropanoate exerts its effects involves interactions with specific molecular targets. The adamantane core can interact with various enzymes and receptors, potentially inhibiting or modulating their activity. The exact pathways and targets depend on the specific application and the modifications made to the compound.
Comparison with Similar Compounds
Similar Compounds
- N-[2-(adamantan-2-yl)ethyl]-N’-R-ureas and -thioureas
- 2-(adamantan-2-yl)ethanamine derivatives
- Adamantane-containing amines and diamines
Uniqueness
Ethyl 2-(adamantan-2-yl)-3-aminopropanoate is unique due to its specific structure, which combines the stability of the adamantane core with the reactivity of the amino and ester groups. This combination allows for a wide range of chemical modifications and applications, making it a versatile compound in scientific research and industrial applications.
Properties
Molecular Formula |
C15H25NO2 |
---|---|
Molecular Weight |
251.36 g/mol |
IUPAC Name |
ethyl 2-(2-adamantyl)-3-aminopropanoate |
InChI |
InChI=1S/C15H25NO2/c1-2-18-15(17)13(8-16)14-11-4-9-3-10(6-11)7-12(14)5-9/h9-14H,2-8,16H2,1H3 |
InChI Key |
YSJUVHYJPMWUPD-UHFFFAOYSA-N |
Canonical SMILES |
CCOC(=O)C(CN)C1C2CC3CC(C2)CC1C3 |
Origin of Product |
United States |
Disclaimer and Information on In-Vitro Research Products
Please be aware that all articles and product information presented on BenchChem are intended solely for informational purposes. The products available for purchase on BenchChem are specifically designed for in-vitro studies, which are conducted outside of living organisms. In-vitro studies, derived from the Latin term "in glass," involve experiments performed in controlled laboratory settings using cells or tissues. It is important to note that these products are not categorized as medicines or drugs, and they have not received approval from the FDA for the prevention, treatment, or cure of any medical condition, ailment, or disease. We must emphasize that any form of bodily introduction of these products into humans or animals is strictly prohibited by law. It is essential to adhere to these guidelines to ensure compliance with legal and ethical standards in research and experimentation.