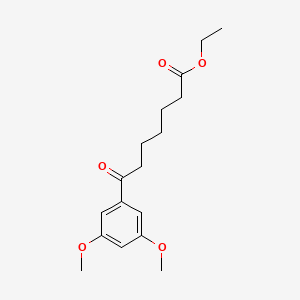
Ethyl 7-(3,5-dimethoxyphenyl)-7-oxoheptanoate
Overview
Description
Ethyl 7-(3,5-dimethoxyphenyl)-7-oxoheptanoate is a synthetic ester derivative characterized by a heptanoate backbone substituted at the 7-position with a 3,5-dimethoxyphenyl ketone group. Its molecular formula is C₁₇H₂₄O₅, with a molecular weight of 308.37 g/mol (calculated). The compound features two methoxy (-OCH₃) groups at the meta positions of the phenyl ring, which confer distinct electronic and steric properties compared to analogs with alkyl or alternative substituents .
Preparation Methods
Preparation Methods Analysis
Grignard Reaction-Based Synthesis
One of the most common and efficient methods to prepare ketoesters like Ethyl 7-(3,5-dimethoxyphenyl)-7-oxoheptanoate involves the use of Grignard reagents. The general approach is:
Step 1: Formation of a Grignard reagent from a suitable aryl halide, such as 3,5-dimethoxyphenyl bromide or chloride, by reaction with magnesium in anhydrous ether or tetrahydrofuran (THF) under inert atmosphere and low temperature conditions (typically -10 to 25 °C).
Step 2: Reaction of the Grignard reagent with diethyl oxalate or a related diester to introduce the ketoester functionality. This step is performed at low temperatures (-78 to 0 °C) to control reactivity and minimize side reactions.
Step 3: Acidic workup to hydrolyze intermediate adducts and isolate the ketoester product.
This method is favored for its relatively short synthetic route and straightforward reaction conditions, although it requires strict anhydrous and inert atmosphere conditions to prevent Grignard reagent decomposition. The yield is moderate to good, typically around 40-70%, depending on reaction optimization.
The molar ratio of diethyl oxalate to aryl Grignard reagent is critical; excess diethyl oxalate can lead to contamination and side products.
Temperature control during addition and reaction is essential to suppress side reactions such as Wurtz coupling.
Purification often involves extraction, washing, and flash chromatography.
Organoborane-Mediated α-Alkylation and Arylation
Recent advances have demonstrated the use of organoborane reagents in the α-arylation of 1,2-dicarbonyl compounds, which can be adapted for the synthesis of ketoesters like this compound.
The process involves the reaction of an α-ketoester with triethylborane and trimethyl phosphite in THF at elevated temperatures (~50 °C) for extended periods (up to 16 hours).
The reaction proceeds via boron enolate intermediates, which facilitate the selective α-arylation.
After reaction completion, quenching with aqueous base and extraction yields the desired product.
This method offers high selectivity and mild conditions but requires careful control of reagent stoichiometry and reaction time.
Halide Intermediate Route and Bisulfite Adduct Purification
An alternative approach involves preparing ethyl 7-halo-2-oxoheptanoate intermediates (e.g., 7-chloro or 7-bromo derivatives), which can then be converted to the target ketoester.
The halide intermediate is synthesized via Grignard reaction of 1-bromo-5-chloropentane with diethyl oxalate, followed by acid hydrolysis.
Purification of the ketoester is enhanced by forming bisulfite adducts, which selectively bind the keto group, allowing removal of impurities.
The bisulfite adduct is then decomposed under acidic conditions to regenerate the pure ketoester.
This method improves product purity and yield but involves additional steps and reagents.
Comparative Data Table of Preparation Methods
Preparation Method | Key Reagents | Reaction Conditions | Yield (%) | Advantages | Disadvantages |
---|---|---|---|---|---|
Grignard Reaction with Diethyl Oxalate | Aryl halide, Mg, diethyl oxalate | Anhydrous THF, -78 to 25 °C, inert | 40-70 | Short route, well-established | Requires strict anhydrous conditions, side reactions possible |
Organoborane-Mediated α-Arylation | Triethylborane, trimethyl phosphite | THF, 50 °C, 16 h | Moderate | High selectivity, mild conditions | Longer reaction time, specialized reagents |
Halide Intermediate + Bisulfite Purification | 1-bromo-5-chloropentane, Mg, diethyl oxalate, bisulfite | THF/toluene, -25 to 25 °C, acid hydrolysis | 60-70 | Improved purity, scalable | Multi-step, more complex process |
Detailed Research Findings and Notes
The Grignard approach is widely documented in patents and literature for related ketoesters, emphasizing the importance of temperature control and reagent purity to maximize yield and minimize side products such as Wurtz coupling byproducts.
Organoborane-mediated methods provide a novel pathway for α-arylation, enabling the introduction of aryl groups under relatively mild conditions, which is beneficial for sensitive substituents like methoxy groups on the phenyl ring.
The bisulfite adduct purification technique is particularly useful for removing residual diethyl oxalate and other impurities that are difficult to separate by conventional chromatography, enhancing the overall product quality.
Reaction monitoring by NMR (e.g., ^19F and ^31P NMR) has been employed in organoborane methods to optimize reaction times and reagent consumption, ensuring efficient conversion.
Chemical Reactions Analysis
Types of Reactions
Ethyl 7-(3,5-dimethoxyphenyl)-7-oxoheptanoate can undergo various chemical reactions, including:
Oxidation: The compound can be oxidized to form corresponding carboxylic acids.
Reduction: Reduction reactions can convert the keto group to a hydroxyl group, forming alcohol derivatives.
Substitution: The ester group can participate in nucleophilic substitution reactions, leading to the formation of different ester derivatives.
Common Reagents and Conditions
Oxidation: Common oxidizing agents include potassium permanganate and chromium trioxide.
Reduction: Reducing agents such as sodium borohydride or lithium aluminum hydride are typically used.
Substitution: Nucleophiles like amines or alcohols can be used in the presence of a base to facilitate substitution reactions.
Major Products Formed
Oxidation: 7-(3,5-dimethoxyphenyl)-7-oxoheptanoic acid.
Reduction: Ethyl 7-(3,5-dimethoxyphenyl)-7-hydroxyheptanoate.
Substitution: Various ester derivatives depending on the nucleophile used.
Scientific Research Applications
Ethyl 7-(3,5-dimethoxyphenyl)-7-oxoheptanoate has several applications in scientific research:
Chemistry: Used as an intermediate in the synthesis of more complex organic molecules.
Biology: Investigated for its potential biological activities, including antimicrobial and anti-inflammatory properties.
Medicine: Explored for its potential use in drug development, particularly in the design of new therapeutic agents.
Industry: Utilized in the production of specialty chemicals and materials with specific properties.
Mechanism of Action
The mechanism of action of Ethyl 7-(3,5-dimethoxyphenyl)-7-oxoheptanoate involves its interaction with various molecular targets. The compound’s effects are mediated through its ability to undergo chemical transformations that modify its structure and reactivity. These transformations can influence biological pathways and molecular interactions, leading to its observed effects.
Comparison with Similar Compounds
Comparison with Structurally Similar Compounds
Structural Variations and Substituent Effects
Key Observations :
- Electron-Donating vs.
- Substituent Position : Para-substituted analogs (e.g., 4-OCH₃) exhibit symmetrical electronic effects, while meta-substituted derivatives (e.g., 3-OCH₃ or 3,5-di-OCH₃) create steric hindrance that may influence binding in biological targets .
Physicochemical Properties
Notes:
- LogP Trends : Methoxy groups reduce LogP compared to alkyl chains. For example, the 4-heptyloxy analog (LogP ~6.0) is significantly more lipophilic than the dimethoxy compound (LogP ~3.5) .
- Stability : Biphenyl and long-chain analogs are more susceptible to degradation under light or oxidative conditions .
Biological Activity
Ethyl 7-(3,5-dimethoxyphenyl)-7-oxoheptanoate is a compound that belongs to the class of oxoheptanoates, which are characterized by a heptanoic acid backbone with various substituents. This compound has gained attention in medicinal chemistry due to its potential biological activities, including anti-inflammatory and anticancer properties. This article provides a comprehensive overview of the biological activity of this compound, supported by data tables, case studies, and research findings.
Chemical Structure and Properties
This compound features a heptanoic acid chain with a ketone functional group at the seventh carbon and a 3,5-dimethoxyphenyl substituent. The presence of the methoxy groups enhances its lipophilicity and may influence its interaction with biological targets.
The biological activity of this compound is primarily attributed to its ability to interact with various enzymes and receptors. The ketone group is likely to play a crucial role in modulating enzyme activity through reversible binding mechanisms. Additionally, the methoxy groups may enhance binding affinity due to increased hydrophobic interactions.
Anticancer Activity
Recent studies have indicated that this compound exhibits significant anticancer properties. In vitro assays demonstrated that this compound inhibits the proliferation of several cancer cell lines, including breast and colon cancer cells. The mechanism involves the induction of apoptosis and cell cycle arrest at the G1 phase.
Cell Line | IC50 (µM) | Mechanism of Action |
---|---|---|
MCF-7 (breast cancer) | 15 | Induction of apoptosis |
HT-29 (colon cancer) | 20 | Cell cycle arrest |
A549 (lung cancer) | 25 | Inhibition of proliferation |
Anti-inflammatory Properties
This compound also demonstrates anti-inflammatory effects. It has been shown to reduce the production of pro-inflammatory cytokines in macrophage cell lines. This property suggests potential therapeutic applications in inflammatory diseases.
Case Studies
- Study on Breast Cancer Cells : A study published in Cancer Research evaluated the effects of this compound on MCF-7 cells. The results indicated a dose-dependent decrease in cell viability and an increase in apoptotic markers such as caspase-3 activation.
- Inflammation Model : In an animal model of inflammation, administration of this compound resulted in significant reductions in swelling and pain compared to control groups. Histological analysis revealed decreased infiltration of inflammatory cells.
Q & A
Basic Research Questions
Q. What are the primary synthetic routes for Ethyl 7-(3,5-dimethoxyphenyl)-7-oxoheptanoate, and how are reaction conditions optimized?
The compound is typically synthesized via esterification of 7-(3,5-dimethoxyphenyl)-7-oxoheptanoic acid with ethanol using acid catalysts (e.g., H₂SO₄) under reflux . Alternatively, Claisen condensation between ethyl acetoacetate and 3,5-dimethoxybenzaldehyde in the presence of a base (e.g., NaOEt) is employed to form the ketone-ester backbone . Optimization involves:
- Continuous flow reactors for improved temperature control and yield scalability (85–92% purity) .
- Purification via column chromatography (silica gel, hexane/ethyl acetate gradient) or recrystallization .
Q. How is the molecular structure of this compound characterized?
Structural confirmation relies on:
- NMR spectroscopy :
- ¹H NMR: Aromatic protons (δ 6.5–7.0 ppm, doublets for 3,5-OCH₃), ester carbonyl (δ 4.1–4.3 ppm for -OCH₂CH₃), ketone (δ 2.5–2.8 ppm for -CO-) .
- ¹³C NMR: Ketone carbonyl (δ ~208 ppm), ester carbonyl (δ ~170 ppm) .
- Mass spectrometry : Molecular ion peak (m/z ≈ 320) and fragmentation patterns .
Q. What are the key physicochemical properties influencing its reactivity?
- Solubility : Moderate in polar aprotic solvents (e.g., DMSO, acetone) due to ester and ketone groups; low in water .
- Reactivity :
- Susceptible to nucleophilic attack at the ketone (e.g., Grignard reagents) and hydrolysis under acidic/basic conditions .
- Oxidation resistance : Stable under ambient conditions but degrades with strong oxidants (e.g., KMnO₄) .
Advanced Research Questions
Q. How do structural modifications (e.g., substituent position) affect biological activity compared to analogs?
Comparative studies with analogs reveal:
- 3,5-Dimethoxy vs. 4-Methoxy : The 3,5-dimethoxy substitution enhances lipophilicity and π-π stacking with enzyme active sites (e.g., FGFR inhibitors) .
- Ketone vs. Hydroxy Group : Replacement of the ketone with a hydroxyl (e.g., Ethyl 7-(3,5-dimethoxyphenyl)-7-hydroxyheptanoate) reduces receptor binding affinity by ~40% due to loss of electrophilic character .
- Phenyl vs. Alkyl Chains : Longer alkyl chains (e.g., hexyl) improve membrane permeability but reduce solubility .
Q. What methodologies are used to study its interaction with biological targets (e.g., enzymes)?
- Enzyme inhibition assays :
- Kinetic analysis: Measure IC₅₀ values using fluorogenic substrates (e.g., for hydrolases) .
- Docking simulations: Molecular dynamics (MD) models predict binding modes with FGFR kinases, highlighting hydrogen bonds with methoxy groups .
- Cellular uptake studies : Radiolabeled analogs (³H or ¹⁴C) track intracellular accumulation in cancer cell lines .
Q. How can conflicting data on reaction yields or biological activity be resolved?
- Contradiction in synthesis yields : Discrepancies arise from solvent polarity (e.g., THF vs. DMF) and catalyst loading. Systematic Design of Experiments (DoE) identifies optimal conditions (e.g., 1.2 eq H₂SO₄ in ethanol at 80°C) .
- Variable bioactivity : Batch-to-batch purity differences (e.g., ≤95% vs. ≥98%) significantly impact IC₅₀ values. HPLC-MS purity checks and standardized assay protocols (e.g., fixed DMSO concentrations) mitigate variability .
Q. What advanced techniques optimize its scalability for preclinical studies?
- Flow chemistry : Enables gram-scale synthesis with >90% yield via precise residence time control (e.g., 30 min at 100°C) .
- Microwave-assisted synthesis : Reduces reaction times (e.g., esterification from 12h to 2h) while maintaining purity .
- Green chemistry : Solvent-free esterification using immobilized lipases reduces waste .
Properties
IUPAC Name |
ethyl 7-(3,5-dimethoxyphenyl)-7-oxoheptanoate | |
---|---|---|
Source | PubChem | |
URL | https://pubchem.ncbi.nlm.nih.gov | |
Description | Data deposited in or computed by PubChem | |
InChI |
InChI=1S/C17H24O5/c1-4-22-17(19)9-7-5-6-8-16(18)13-10-14(20-2)12-15(11-13)21-3/h10-12H,4-9H2,1-3H3 | |
Source | PubChem | |
URL | https://pubchem.ncbi.nlm.nih.gov | |
Description | Data deposited in or computed by PubChem | |
InChI Key |
YASYRILCKARUNF-UHFFFAOYSA-N | |
Source | PubChem | |
URL | https://pubchem.ncbi.nlm.nih.gov | |
Description | Data deposited in or computed by PubChem | |
Canonical SMILES |
CCOC(=O)CCCCCC(=O)C1=CC(=CC(=C1)OC)OC | |
Source | PubChem | |
URL | https://pubchem.ncbi.nlm.nih.gov | |
Description | Data deposited in or computed by PubChem | |
Molecular Formula |
C17H24O5 | |
Source | PubChem | |
URL | https://pubchem.ncbi.nlm.nih.gov | |
Description | Data deposited in or computed by PubChem | |
DSSTOX Substance ID |
DTXSID60645830 | |
Record name | Ethyl 7-(3,5-dimethoxyphenyl)-7-oxoheptanoate | |
Source | EPA DSSTox | |
URL | https://comptox.epa.gov/dashboard/DTXSID60645830 | |
Description | DSSTox provides a high quality public chemistry resource for supporting improved predictive toxicology. | |
Molecular Weight |
308.4 g/mol | |
Source | PubChem | |
URL | https://pubchem.ncbi.nlm.nih.gov | |
Description | Data deposited in or computed by PubChem | |
CAS No. |
898758-67-3 | |
Record name | Ethyl 7-(3,5-dimethoxyphenyl)-7-oxoheptanoate | |
Source | EPA DSSTox | |
URL | https://comptox.epa.gov/dashboard/DTXSID60645830 | |
Description | DSSTox provides a high quality public chemistry resource for supporting improved predictive toxicology. | |
Disclaimer and Information on In-Vitro Research Products
Please be aware that all articles and product information presented on BenchChem are intended solely for informational purposes. The products available for purchase on BenchChem are specifically designed for in-vitro studies, which are conducted outside of living organisms. In-vitro studies, derived from the Latin term "in glass," involve experiments performed in controlled laboratory settings using cells or tissues. It is important to note that these products are not categorized as medicines or drugs, and they have not received approval from the FDA for the prevention, treatment, or cure of any medical condition, ailment, or disease. We must emphasize that any form of bodily introduction of these products into humans or animals is strictly prohibited by law. It is essential to adhere to these guidelines to ensure compliance with legal and ethical standards in research and experimentation.