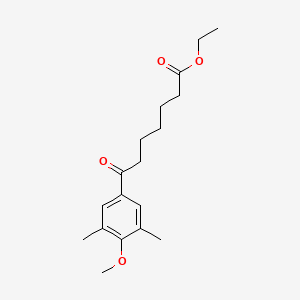
Ethyl 7-(3,5-dimethyl-4-methoxyphenyl)-7-oxoheptanoate
- Click on QUICK INQUIRY to receive a quote from our team of experts.
- With the quality product at a COMPETITIVE price, you can focus more on your research.
Overview
Description
The compound “Ethyl 7-(3,5-dimethyl-4-methoxyphenyl)-7-oxoheptanoate” is an ester, which is a class of organic compounds that react with water to produce alcohols and organic or inorganic acids . Esters are commonly used in a wide variety of applications from plastics to perfumes.
Synthesis Analysis
The synthesis of such a compound would likely involve the reaction of an alcohol with a carboxylic acid in the presence of a catalyst . The specific reactants would depend on the exact structure of the desired product.Molecular Structure Analysis
The molecular structure of this compound would likely include a seven-carbon chain (heptanoate) attached to an ethyl group at one end and a 3,5-dimethyl-4-methoxyphenyl group at the other .Chemical Reactions Analysis
As an ester, this compound would be expected to undergo reactions typical of this class of compounds. This could include hydrolysis to form an alcohol and a carboxylic acid .Physical And Chemical Properties Analysis
The physical and chemical properties of a compound can be influenced by its molecular structure. For example, the presence of the ester group in this compound could influence its solubility, boiling point, and reactivity .Scientific Research Applications
Understanding Chemical Interactions
The chemical structure of mescaline and its analogs, including compounds similar to Ethyl 7-(3,5-dimethyl-4-methoxyphenyl)-7-oxoheptanoate, have been studied for their toxicity and behavior in laboratory animals. These studies explore how structural modifications, like alpha substitution or introduction of specific functional groups, can significantly alter pharmacological activity. This research is critical in understanding the toxicological and behavioral responses in different species, shedding light on the complex interplay between chemical structure and biological function (Hardman, Haavik, & Seevers, 1973).
Biodegradation and Environmental Impact
Research into compounds like Ethyl tert-butyl ether (ETBE), structurally similar to Ethyl 7-(3,5-dimethyl-4-methoxyphenyl)-7-oxoheptanoate, highlights the importance of understanding the biodegradation pathways and environmental fate of chemical substances. Microorganisms capable of degrading ETBE, including their metabolic pathways and limitations, are extensively studied. This research is pivotal in assessing and mitigating the environmental impact of these substances, guiding strategies for bioremediation and environmental conservation (Thornton et al., 2020).
Advanced Materials and Polymer Research
The exploration of compounds like Ethyl 7-(3,5-dimethyl-4-methoxyphenyl)-7-oxoheptanoate is not limited to toxicological studies. These compounds play a significant role in the development of advanced materials. For instance, the study of methylene-linked liquid crystal dimers, which share structural similarities, contributes to our understanding of materials science, particularly in the creation of new nematic phases with unique properties. This research has implications for the development of advanced display technologies and other applications in materials science (Henderson & Imrie, 2011).
Safety and Hazards
properties
IUPAC Name |
ethyl 7-(4-methoxy-3,5-dimethylphenyl)-7-oxoheptanoate |
Source
|
---|---|---|
Source | PubChem | |
URL | https://pubchem.ncbi.nlm.nih.gov | |
Description | Data deposited in or computed by PubChem | |
InChI |
InChI=1S/C18H26O4/c1-5-22-17(20)10-8-6-7-9-16(19)15-11-13(2)18(21-4)14(3)12-15/h11-12H,5-10H2,1-4H3 |
Source
|
Source | PubChem | |
URL | https://pubchem.ncbi.nlm.nih.gov | |
Description | Data deposited in or computed by PubChem | |
InChI Key |
ZKRSPCNGQCSLBQ-UHFFFAOYSA-N |
Source
|
Source | PubChem | |
URL | https://pubchem.ncbi.nlm.nih.gov | |
Description | Data deposited in or computed by PubChem | |
Canonical SMILES |
CCOC(=O)CCCCCC(=O)C1=CC(=C(C(=C1)C)OC)C |
Source
|
Source | PubChem | |
URL | https://pubchem.ncbi.nlm.nih.gov | |
Description | Data deposited in or computed by PubChem | |
Molecular Formula |
C18H26O4 |
Source
|
Source | PubChem | |
URL | https://pubchem.ncbi.nlm.nih.gov | |
Description | Data deposited in or computed by PubChem | |
DSSTOX Substance ID |
DTXSID80645534 |
Source
|
Record name | Ethyl 7-(4-methoxy-3,5-dimethylphenyl)-7-oxoheptanoate | |
Source | EPA DSSTox | |
URL | https://comptox.epa.gov/dashboard/DTXSID80645534 | |
Description | DSSTox provides a high quality public chemistry resource for supporting improved predictive toxicology. | |
Molecular Weight |
306.4 g/mol |
Source
|
Source | PubChem | |
URL | https://pubchem.ncbi.nlm.nih.gov | |
Description | Data deposited in or computed by PubChem | |
Product Name |
Ethyl 7-(3,5-dimethyl-4-methoxyphenyl)-7-oxoheptanoate | |
CAS RN |
898751-10-5 |
Source
|
Record name | Ethyl 7-(4-methoxy-3,5-dimethylphenyl)-7-oxoheptanoate | |
Source | EPA DSSTox | |
URL | https://comptox.epa.gov/dashboard/DTXSID80645534 | |
Description | DSSTox provides a high quality public chemistry resource for supporting improved predictive toxicology. | |
Disclaimer and Information on In-Vitro Research Products
Please be aware that all articles and product information presented on BenchChem are intended solely for informational purposes. The products available for purchase on BenchChem are specifically designed for in-vitro studies, which are conducted outside of living organisms. In-vitro studies, derived from the Latin term "in glass," involve experiments performed in controlled laboratory settings using cells or tissues. It is important to note that these products are not categorized as medicines or drugs, and they have not received approval from the FDA for the prevention, treatment, or cure of any medical condition, ailment, or disease. We must emphasize that any form of bodily introduction of these products into humans or animals is strictly prohibited by law. It is essential to adhere to these guidelines to ensure compliance with legal and ethical standards in research and experimentation.