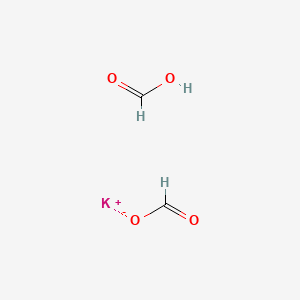
Potassium diformate
Overview
Description
Potassium diformate is a chemical compound with the molecular formula C2H3KO4. It is a white or slightly yellow crystalline powder that is odorless and non-corrosive. This compound is primarily used as a feed additive in animal nutrition, particularly in swine diets, due to its antimicrobial properties and ability to promote growth. It is the first non-antibiotic feed additive approved by the European Union as an alternative to antibiotic growth promoters .
Mechanism of Action
Target of Action
Potassium diformate (KDF) is primarily targeted towards the gut microbiota of animals, particularly in aquatic organisms . It is used as a feed additive and has been shown to have a significant impact on the growth and reproduction of these organisms . The primary targets of KDF are harmful bacteria such as E. coli and Salmonella .
Mode of Action
The action mechanism of KDF is mainly the action of small organic acid formic acid and potassium ion . Formate anions, derived from KDF, break down bacterial cell wall proteins outside the cell wall, playing a bactericidal and reducing role in bacteria such as E. coli and Salmonella . This antimicrobial effect reduces the general bacterial population in the gut, especially harmful bacteria, and promotes a more favorable microflora in the gut .
Biochemical Pathways
KDF affects several biochemical pathways. It has been shown to significantly reduce levels of alanine aminotransferase (ALT), aspartate aminotransferase (AST), alkaline phosphatase (ALP), and lactate dehydrogenase (LDH) enzymes, and increase serum total protein, globulin, albumin, lysozyme enzyme activity, and alternative complement pathway activity . Moreover, KDF significantly increases digestive enzyme activities (protease, trypsin, chymotrypsin, lipase, and alpha amylase), with the highest levels seen in certain dosage groups .
Result of Action
The use of KDF results in improved growth performance parameters in animals, such as Beluga fish . It also improves digestion and reduces the pH of the gastrointestinal tract of chickens infected with S. pullorum . Furthermore, KDF has been shown to significantly modify the diversity and abundance of intestinal microflora in animals . In particular, it promotes the colonization of several probiotics, which are involved in maintenance of the intestinal barrier, modulation of inflammation, energy supply for intestinal cells, and pathogen resistance .
Action Environment
The action of KDF can be influenced by environmental factors. For instance, the use of KDF is less frequently reported in industrially underdeveloped areas . Moreover, the effectiveness of KDF can be influenced by the diet of the organism, as well as the presence of other compounds. For example, a study found that combining KDF with butyric acid resulted in improved growth and enhanced immune system in Beluga fish .
Biochemical Analysis
Biochemical Properties
Potassium diformate plays a significant role in biochemical reactions by acting as an acidifier. It interacts with various enzymes, proteins, and other biomolecules. For instance, this compound has been shown to reduce levels of enzymes such as alanine aminotransferase, aspartate aminotransferase, alkaline phosphatase, and lactate dehydrogenase . Additionally, it increases the activity of digestive enzymes like protease, trypsin, chymotrypsin, lipase, and alpha-amylase . These interactions suggest that this compound can modulate enzyme activity and improve metabolic processes.
Cellular Effects
This compound influences various types of cells and cellular processes. It has been observed to enhance the immune response by increasing serum total protein, globulin, albumin, lysozyme enzyme activity, and alternative complement pathway activity . Furthermore, this compound positively impacts cell signaling pathways, gene expression, and cellular metabolism. For example, it has been shown to improve growth performance and reduce stress markers in juvenile Beluga fish .
Molecular Mechanism
The molecular mechanism of this compound involves its interaction with biomolecules, leading to enzyme inhibition or activation and changes in gene expression. This compound binds to specific enzymes, modulating their activity and influencing metabolic pathways. It also affects the expression of genes related to immune response and stress regulation . These molecular interactions contribute to the overall beneficial effects of this compound on cellular function.
Temporal Effects in Laboratory Settings
In laboratory settings, the effects of this compound change over time. The compound’s stability and degradation can influence its long-term effects on cellular function. Studies have shown that this compound maintains its efficacy over extended periods, leading to sustained improvements in growth performance and immune response . The long-term effects on cellular function may vary depending on the specific conditions and duration of exposure.
Dosage Effects in Animal Models
The effects of this compound vary with different dosages in animal models. Lower doses of this compound have been shown to promote growth and improve biochemical parameters, while higher doses may lead to adverse effects . For example, a dosage of 0.15% this compound for 30 days was found to be most effective in promoting growth in juvenile Beluga fish . Higher doses may result in toxicity and negatively impact cellular function.
Metabolic Pathways
This compound is involved in various metabolic pathways, interacting with enzymes and cofactors. It modulates metabolic flux and affects metabolite levels. For instance, this compound has been shown to increase the activity of digestive enzymes, leading to improved nutrient absorption and metabolism . These effects on metabolic pathways contribute to the overall health benefits of this compound.
Transport and Distribution
This compound is transported and distributed within cells and tissues through specific transporters and binding proteins. These interactions influence its localization and accumulation in different cellular compartments. The compound’s distribution within the body can affect its efficacy and overall impact on cellular function .
Subcellular Localization
The subcellular localization of this compound plays a crucial role in its activity and function. The compound may be directed to specific compartments or organelles through targeting signals or post-translational modifications. These localization mechanisms ensure that this compound exerts its effects in the appropriate cellular context .
Preparation Methods
Synthetic Routes and Reaction Conditions: Potassium diformate is synthesized by reacting formic acid with potassium hydroxide or potassium carbonate. The reaction typically occurs at temperatures ranging from 45 to 80 degrees Celsius for 2 to 6 hours. The resulting solution is then concentrated under vacuum at 65 to 75 degrees Celsius until the volume is reduced to about half or two-thirds of the initial volume. The concentrated solution is cooled to 20 degrees Celsius for crystallization, which takes 20 to 28 hours .
Industrial Production Methods: In industrial settings, this compound is produced using similar methods but on a larger scale. The process involves the use of high-purity formic acid and potassium hydroxide or potassium carbonate. The reaction conditions are carefully controlled to ensure high yield and purity of the final product. The crystallized this compound is then dried and packaged for use as a feed additive .
Chemical Reactions Analysis
Types of Reactions: Potassium diformate undergoes several types of chemical reactions, including:
Oxidation: this compound can be oxidized to produce carbon dioxide and water.
Reduction: It can be reduced to formic acid and potassium formate.
Substitution: this compound can participate in substitution reactions where the formate ion is replaced by other anions.
Common Reagents and Conditions:
Oxidation: Typically involves the use of oxidizing agents such as potassium permanganate or hydrogen peroxide under acidic conditions.
Reduction: Can be achieved using reducing agents like sodium borohydride or lithium aluminum hydride.
Substitution: Often involves the use of halogenated compounds or other reactive anions under controlled conditions.
Major Products Formed:
Oxidation: Carbon dioxide and water.
Reduction: Formic acid and potassium formate.
Substitution: Various substituted formate compounds depending on the reacting anion.
Scientific Research Applications
Potassium diformate has a wide range of applications in scientific research, including:
Chemistry: Used as a reagent in various chemical reactions and as a buffer in analytical chemistry.
Biology: Employed in studies related to microbial growth inhibition and gut microbiota modulation.
Medicine: Investigated for its potential antimicrobial properties and its role in enhancing nutrient absorption.
Industry: Widely used as a feed additive in animal nutrition to promote growth and improve feed efficiency. .
Comparison with Similar Compounds
Potassium diformate is often compared with other organic acid salts used as feed additives, such as:
Butyric Acid: Known for its role in gut health and immune modulation. Unlike this compound, butyric acid has a more pronounced effect on the gut lining and immune response.
Calcium Diformate: Similar to this compound but with calcium as the cation. It is used for similar purposes but may have different solubility and bioavailability properties.
Sodium Formate: Another formate salt used in various industrial applications. It is less commonly used as a feed additive compared to this compound.
Uniqueness of this compound: this compound stands out due to its dual action of formic acid and formate, providing both antimicrobial effects and growth promotion. Its approval by the European Union as a non-antibiotic growth promoter highlights its safety and efficacy in animal nutrition .
Properties
IUPAC Name |
potassium;formic acid;formate | |
---|---|---|
Source | PubChem | |
URL | https://pubchem.ncbi.nlm.nih.gov | |
Description | Data deposited in or computed by PubChem | |
InChI |
InChI=1S/2CH2O2.K/c2*2-1-3;/h2*1H,(H,2,3);/q;;+1/p-1 | |
Source | PubChem | |
URL | https://pubchem.ncbi.nlm.nih.gov | |
Description | Data deposited in or computed by PubChem | |
InChI Key |
BINNZIDCJWQYOH-UHFFFAOYSA-M | |
Source | PubChem | |
URL | https://pubchem.ncbi.nlm.nih.gov | |
Description | Data deposited in or computed by PubChem | |
Canonical SMILES |
C(=O)O.C(=O)[O-].[K+] | |
Source | PubChem | |
URL | https://pubchem.ncbi.nlm.nih.gov | |
Description | Data deposited in or computed by PubChem | |
Molecular Formula |
C2H3KO4 | |
Source | PubChem | |
URL | https://pubchem.ncbi.nlm.nih.gov | |
Description | Data deposited in or computed by PubChem | |
DSSTOX Substance ID |
DTXSID2029745 | |
Record name | Potassium formate | |
Source | EPA DSSTox | |
URL | https://comptox.epa.gov/dashboard/DTXSID2029745 | |
Description | DSSTox provides a high quality public chemistry resource for supporting improved predictive toxicology. | |
Molecular Weight |
130.14 g/mol | |
Source | PubChem | |
URL | https://pubchem.ncbi.nlm.nih.gov | |
Description | Data deposited in or computed by PubChem | |
CAS No. |
20642-05-1 | |
Record name | Potassium diformate | |
Source | ChemIDplus | |
URL | https://pubchem.ncbi.nlm.nih.gov/substance/?source=chemidplus&sourceid=0020642051 | |
Description | ChemIDplus is a free, web search system that provides access to the structure and nomenclature authority files used for the identification of chemical substances cited in National Library of Medicine (NLM) databases, including the TOXNET system. | |
Record name | Potassium formate | |
Source | EPA DSSTox | |
URL | https://comptox.epa.gov/dashboard/DTXSID2029745 | |
Description | DSSTox provides a high quality public chemistry resource for supporting improved predictive toxicology. | |
Record name | Potassium formate(1:2) | |
Source | European Chemicals Agency (ECHA) | |
URL | https://echa.europa.eu/substance-information/-/substanceinfo/100.039.925 | |
Description | The European Chemicals Agency (ECHA) is an agency of the European Union which is the driving force among regulatory authorities in implementing the EU's groundbreaking chemicals legislation for the benefit of human health and the environment as well as for innovation and competitiveness. | |
Explanation | Use of the information, documents and data from the ECHA website is subject to the terms and conditions of this Legal Notice, and subject to other binding limitations provided for under applicable law, the information, documents and data made available on the ECHA website may be reproduced, distributed and/or used, totally or in part, for non-commercial purposes provided that ECHA is acknowledged as the source: "Source: European Chemicals Agency, http://echa.europa.eu/". Such acknowledgement must be included in each copy of the material. ECHA permits and encourages organisations and individuals to create links to the ECHA website under the following cumulative conditions: Links can only be made to webpages that provide a link to the Legal Notice page. | |
Record name | POTASSIUM DIFORMATE | |
Source | FDA Global Substance Registration System (GSRS) | |
URL | https://gsrs.ncats.nih.gov/ginas/app/beta/substances/4FHJ7DIT8M | |
Description | The FDA Global Substance Registration System (GSRS) enables the efficient and accurate exchange of information on what substances are in regulated products. Instead of relying on names, which vary across regulatory domains, countries, and regions, the GSRS knowledge base makes it possible for substances to be defined by standardized, scientific descriptions. | |
Explanation | Unless otherwise noted, the contents of the FDA website (www.fda.gov), both text and graphics, are not copyrighted. They are in the public domain and may be republished, reprinted and otherwise used freely by anyone without the need to obtain permission from FDA. Credit to the U.S. Food and Drug Administration as the source is appreciated but not required. | |
Retrosynthesis Analysis
AI-Powered Synthesis Planning: Our tool employs the Template_relevance Pistachio, Template_relevance Bkms_metabolic, Template_relevance Pistachio_ringbreaker, Template_relevance Reaxys, Template_relevance Reaxys_biocatalysis model, leveraging a vast database of chemical reactions to predict feasible synthetic routes.
One-Step Synthesis Focus: Specifically designed for one-step synthesis, it provides concise and direct routes for your target compounds, streamlining the synthesis process.
Accurate Predictions: Utilizing the extensive PISTACHIO, BKMS_METABOLIC, PISTACHIO_RINGBREAKER, REAXYS, REAXYS_BIOCATALYSIS database, our tool offers high-accuracy predictions, reflecting the latest in chemical research and data.
Strategy Settings
Precursor scoring | Relevance Heuristic |
---|---|
Min. plausibility | 0.01 |
Model | Template_relevance |
Template Set | Pistachio/Bkms_metabolic/Pistachio_ringbreaker/Reaxys/Reaxys_biocatalysis |
Top-N result to add to graph | 6 |
Feasible Synthetic Routes
Disclaimer and Information on In-Vitro Research Products
Please be aware that all articles and product information presented on BenchChem are intended solely for informational purposes. The products available for purchase on BenchChem are specifically designed for in-vitro studies, which are conducted outside of living organisms. In-vitro studies, derived from the Latin term "in glass," involve experiments performed in controlled laboratory settings using cells or tissues. It is important to note that these products are not categorized as medicines or drugs, and they have not received approval from the FDA for the prevention, treatment, or cure of any medical condition, ailment, or disease. We must emphasize that any form of bodily introduction of these products into humans or animals is strictly prohibited by law. It is essential to adhere to these guidelines to ensure compliance with legal and ethical standards in research and experimentation.