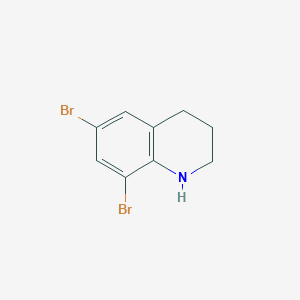
6,8-Dibromo-1,2,3,4-tetrahydroquinoline
Overview
Description
6,8-Dibromo-1,2,3,4-tetrahydroquinoline is a brominated derivative of tetrahydroquinoline, a compound that forms the backbone of many pharmacologically active molecules. The presence of bromine atoms at the 6 and 8 positions of the quinoline ring enhances its reactivity and potential for further functionalization . This compound is of significant interest in organic chemistry due to its versatile applications in the synthesis of various biologically active molecules .
Mechanism of Action
Target of Action
Quinoline derivatives, which include 6,8-dibromo-1,2,3,4-tetrahydroquinoline, are known for their wide range of biological activities and chemotherapeutic activities .
Mode of Action
The mode of action of this compound involves electrophilic bromination and radical dehydrogenation sequences . This compound is synthesized from tetrahydroquinolines using NBS as the electrophile and oxidant . The cascade transformation proceeds with good functional group tolerance under metal-free conditions .
Biochemical Pathways
Quinoline derivatives are known to affect various biochemical pathways, including those involved in cell cycle arrest, apoptosis, disruption of cell migration, inhibition of angiogenesis .
Pharmacokinetics
The compound’s molecular weight is 290982460737228 , which could influence its pharmacokinetic properties.
Biochemical Analysis
Biochemical Properties
6,8-Dibromo-1,2,3,4-tetrahydroquinoline plays a significant role in biochemical reactions, particularly in the context of its antiproliferative activity. This compound has been shown to interact with several enzymes and proteins, influencing their activity. For instance, it has been observed to cause DNA fragmentation in certain cancer cell lines, indicating its potential to induce apoptosis . It does not inhibit the Topoisomerase I enzyme, which is often targeted by other anticancer agents . The interactions of this compound with these biomolecules highlight its potential as a selective anticancer agent.
Cellular Effects
The effects of this compound on various cell types and cellular processes are profound. This compound has demonstrated antiproliferative activity against several cancer cell lines, including rat glioblastoma (C6), human cervical cancer (HeLa), and human adenocarcinoma (HT29) cells . It induces apoptosis in these cells, leading to cell death. Additionally, this compound influences cell signaling pathways and gene expression, contributing to its anticancer effects . The impact on cellular metabolism and the disruption of cell cycle progression further underscore its potential therapeutic applications.
Molecular Mechanism
At the molecular level, this compound exerts its effects through several mechanisms. One of the primary actions is the induction of apoptosis via DNA fragmentation . This compound interacts with specific biomolecules, leading to the activation of apoptotic pathways. Although it does not inhibit Topoisomerase I, it still effectively induces cell death in cancer cells . The binding interactions and enzyme modulation by this compound are crucial for its antiproliferative activity.
Temporal Effects in Laboratory Settings
The stability and degradation of this compound over time have been studied in laboratory settings. This compound has shown consistent antiproliferative effects in in vitro studies, with its activity remaining stable over extended periods . Long-term exposure to this compound has been associated with sustained antiproliferative effects and apoptosis induction in cancer cell lines . These findings suggest that the compound maintains its efficacy over time, making it a promising candidate for further development.
Dosage Effects in Animal Models
In animal models, the effects of this compound vary with different dosages. Studies have shown that lower doses of the compound can effectively inhibit tumor growth without causing significant toxicity . Higher doses may lead to adverse effects, including toxicity and damage to healthy tissues . The threshold effects observed in these studies highlight the importance of optimizing dosage to maximize therapeutic benefits while minimizing side effects.
Metabolic Pathways
This compound is involved in several metabolic pathways, interacting with various enzymes and cofactors. The compound undergoes metabolic transformations that influence its activity and efficacy . These metabolic pathways are crucial for understanding the compound’s pharmacokinetics and optimizing its therapeutic potential. The interactions with metabolic enzymes also affect the levels of metabolites, contributing to the overall biochemical effects of this compound .
Transport and Distribution
The transport and distribution of this compound within cells and tissues are essential for its biological activity. This compound is transported across cell membranes and distributed to various cellular compartments . It interacts with specific transporters and binding proteins, influencing its localization and accumulation within cells . Understanding these transport mechanisms is vital for optimizing the delivery and efficacy of this compound in therapeutic applications.
Subcellular Localization
The subcellular localization of this compound plays a critical role in its activity and function. This compound is directed to specific cellular compartments, where it exerts its effects . Targeting signals and post-translational modifications influence the localization of this compound, directing it to organelles such as the nucleus and mitochondria . These localization patterns are essential for understanding the compound’s mechanism of action and optimizing its therapeutic potential.
Preparation Methods
Synthetic Routes and Reaction Conditions
The synthesis of 6,8-dibromo-1,2,3,4-tetrahydroquinoline typically involves the bromination of 1,2,3,4-tetrahydroquinoline. One common method is the direct bromination using molecular bromine (Br2) in the presence of a suitable solvent such as acetic acid . The reaction is carried out under controlled conditions to ensure selective bromination at the 6 and 8 positions .
Industrial Production Methods
In an industrial setting, the production of this compound can be scaled up by optimizing the reaction conditions, such as temperature, solvent, and bromine concentration. Continuous flow reactors may be employed to enhance the efficiency and yield of the bromination process .
Chemical Reactions Analysis
Types of Reactions
6,8-Dibromo-1,2,3,4-tetrahydroquinoline undergoes various types of chemical reactions, including:
Substitution Reactions: The bromine atoms can be substituted with other functional groups through nucleophilic substitution reactions.
Oxidation Reactions: The compound can be oxidized to form quinoline N-oxides, which are useful intermediates in organic synthesis.
Reduction Reactions: Reduction of the brominated compound can lead to the formation of tetrahydroquinoline derivatives with different substitution patterns.
Common Reagents and Conditions
Bromination: Molecular bromine (Br2) in acetic acid.
Substitution: Sodium methoxide (NaOMe) and copper(I) iodide (CuI).
Oxidation: Various oxidizing agents such as hydrogen peroxide (H2O2) or peracids.
Major Products
Methoxy Derivatives: Formed through nucleophilic substitution reactions.
Quinoline N-Oxides: Formed through oxidation reactions.
Scientific Research Applications
6,8-Dibromo-1,2,3,4-tetrahydroquinoline has a wide range of applications in scientific research:
Chemistry: Used as a building block for the synthesis of more complex organic molecules.
Biology: Investigated for its potential biological activities, including anticancer properties.
Medicine: Explored as a precursor for the development of pharmacologically active compounds.
Industry: Utilized in the production of specialty chemicals and materials.
Comparison with Similar Compounds
Similar Compounds
6-Bromo-1,2,3,4-tetrahydroquinoline: A mono-brominated derivative with similar reactivity but different substitution patterns.
3,6,8-Tribromoquinoline: A tri-brominated derivative with enhanced reactivity due to the presence of three bromine atoms.
Uniqueness
6,8-Dibromo-1,2,3,4-tetrahydroquinoline is unique due to its specific bromination pattern, which allows for selective functionalization and the formation of diverse derivatives. This makes it a valuable intermediate in the synthesis of complex organic molecules with potential biological activities .
Properties
IUPAC Name |
6,8-dibromo-1,2,3,4-tetrahydroquinoline | |
---|---|---|
Source | PubChem | |
URL | https://pubchem.ncbi.nlm.nih.gov | |
Description | Data deposited in or computed by PubChem | |
InChI |
InChI=1S/C9H9Br2N/c10-7-4-6-2-1-3-12-9(6)8(11)5-7/h4-5,12H,1-3H2 | |
Source | PubChem | |
URL | https://pubchem.ncbi.nlm.nih.gov | |
Description | Data deposited in or computed by PubChem | |
InChI Key |
SNSRBNSPFBIZRO-UHFFFAOYSA-N | |
Source | PubChem | |
URL | https://pubchem.ncbi.nlm.nih.gov | |
Description | Data deposited in or computed by PubChem | |
Canonical SMILES |
C1CC2=C(C(=CC(=C2)Br)Br)NC1 | |
Source | PubChem | |
URL | https://pubchem.ncbi.nlm.nih.gov | |
Description | Data deposited in or computed by PubChem | |
Molecular Formula |
C9H9Br2N | |
Source | PubChem | |
URL | https://pubchem.ncbi.nlm.nih.gov | |
Description | Data deposited in or computed by PubChem | |
Molecular Weight |
290.98 g/mol | |
Source | PubChem | |
URL | https://pubchem.ncbi.nlm.nih.gov | |
Description | Data deposited in or computed by PubChem | |
Retrosynthesis Analysis
AI-Powered Synthesis Planning: Our tool employs the Template_relevance Pistachio, Template_relevance Bkms_metabolic, Template_relevance Pistachio_ringbreaker, Template_relevance Reaxys, Template_relevance Reaxys_biocatalysis model, leveraging a vast database of chemical reactions to predict feasible synthetic routes.
One-Step Synthesis Focus: Specifically designed for one-step synthesis, it provides concise and direct routes for your target compounds, streamlining the synthesis process.
Accurate Predictions: Utilizing the extensive PISTACHIO, BKMS_METABOLIC, PISTACHIO_RINGBREAKER, REAXYS, REAXYS_BIOCATALYSIS database, our tool offers high-accuracy predictions, reflecting the latest in chemical research and data.
Strategy Settings
Precursor scoring | Relevance Heuristic |
---|---|
Min. plausibility | 0.01 |
Model | Template_relevance |
Template Set | Pistachio/Bkms_metabolic/Pistachio_ringbreaker/Reaxys/Reaxys_biocatalysis |
Top-N result to add to graph | 6 |
Feasible Synthetic Routes
Q1: How can 6,8-dibromo-1,2,3,4-tetrahydroquinoline be chemically modified, and what are the implications for its activity?
A: This compound serves as a versatile building block for synthesizing various substituted quinoline derivatives. Researchers, such as those in [], have successfully utilized Suzuki-Miyaura coupling reactions to introduce aryl substituents at the 6- and 8- positions of the molecule. These modifications allow for exploring the structure-activity relationship (SAR) and optimizing the compound's properties for desired applications. By introducing different substituents, researchers can investigate how these changes impact the compound's interaction with biological targets, potentially leading to enhanced potency or selectivity.
Q2: What are the decision-making processes involved in identifying promising anticancer agents based on experimental data, and how does this compound fit into this context?
A: Identifying promising anticancer agents often involves evaluating multiple criteria, including antiproliferation activity and cytotoxicity. Rahimizadeh et al. [] utilized a multi-criteria decision-making methodology (MCDM) alongside experimental data to rank the efficacy of several quinoline derivatives. Their research identified this compound as one of the most promising agents against the tested cancer cell lines. This highlights the importance of combining experimental results with robust analytical tools like MCDM to systematically identify and prioritize lead compounds in drug discovery.
Disclaimer and Information on In-Vitro Research Products
Please be aware that all articles and product information presented on BenchChem are intended solely for informational purposes. The products available for purchase on BenchChem are specifically designed for in-vitro studies, which are conducted outside of living organisms. In-vitro studies, derived from the Latin term "in glass," involve experiments performed in controlled laboratory settings using cells or tissues. It is important to note that these products are not categorized as medicines or drugs, and they have not received approval from the FDA for the prevention, treatment, or cure of any medical condition, ailment, or disease. We must emphasize that any form of bodily introduction of these products into humans or animals is strictly prohibited by law. It is essential to adhere to these guidelines to ensure compliance with legal and ethical standards in research and experimentation.