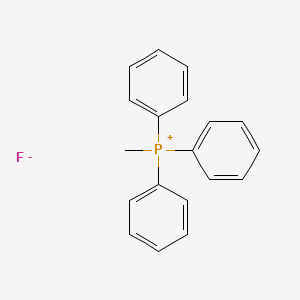
Methyltriphenylphosphanium fluoride
- Click on QUICK INQUIRY to receive a quote from our team of experts.
- With the quality product at a COMPETITIVE price, you can focus more on your research.
Overview
Description
Methyltriphenylphosphanium fluoride is an organophosphorus compound with the chemical formula C19H18FP It is a quaternary phosphonium salt, characterized by the presence of a methyl group attached to a triphenylphosphonium cation, with fluoride as the counterion
Preparation Methods
Synthetic Routes and Reaction Conditions: Methyltriphenylphosphanium fluoride can be synthesized through the reaction of triphenylphosphine with methyl fluoride. The reaction typically involves the use of a strong base to facilitate the nucleophilic substitution reaction. The general reaction scheme is as follows: [ \text{Ph}_3\text{P} + \text{CH}_3\text{F} \rightarrow \text{Ph}_3\text{PCH}_3^+ \text{F}^- ]
Industrial Production Methods: Industrial production of this compound involves similar synthetic routes but on a larger scale. The reaction conditions are optimized to ensure high yield and purity of the product. The use of automated reactors and continuous flow systems can enhance the efficiency of the production process.
Chemical Reactions Analysis
Types of Reactions: Methyltriphenylphosphanium fluoride undergoes various types of chemical reactions, including:
Nucleophilic Substitution: The fluoride ion can act as a nucleophile, participating in substitution reactions.
Oxidation and Reduction: The compound can undergo redox reactions, although these are less common.
Complex Formation: It can form complexes with various metal ions.
Common Reagents and Conditions:
Nucleophilic Substitution: Common reagents include alkyl halides and strong bases such as sodium hydride.
Oxidation: Oxidizing agents like hydrogen peroxide can be used.
Reduction: Reducing agents such as lithium aluminum hydride may be employed.
Major Products Formed:
Substitution Reactions: Products typically include substituted phosphonium salts.
Oxidation and Reduction: Products vary depending on the specific reaction conditions and reagents used.
Scientific Research Applications
Methyltriphenylphosphanium fluoride has a wide range of applications in scientific research:
Chemistry: It is used as a reagent in organic synthesis, particularly in the preparation of fluorinated compounds.
Biology: The compound is utilized in studies involving mitochondrial function due to its ability to accumulate in the mitochondrial matrix.
Medicine: Research is ongoing into its potential use in drug delivery systems, particularly for targeting mitochondria.
Industry: It is used in the production of specialty chemicals and materials, including fluorinated polymers.
Mechanism of Action
The mechanism by which methyltriphenylphosphanium fluoride exerts its effects is primarily through its interaction with cellular components. The triphenylphosphonium moiety allows the compound to accumulate in the mitochondrial matrix, where it can affect mitochondrial function. Specifically, it has been shown to inhibit the Krebs cycle enzyme 2-oxoglutarate dehydrogenase, leading to alterations in cellular respiration and energy production .
Comparison with Similar Compounds
Methyltriphenylphosphonium Bromide: Similar in structure but with bromide as the counterion. It is also used in organic synthesis and has similar applications.
Tetraphenylphosphonium Chloride: Another quaternary phosphonium salt with chloride as the counterion. It is used in various chemical reactions and studies involving cellular function.
Uniqueness: Methyltriphenylphosphanium fluoride is unique due to the presence of the fluoride ion, which imparts distinct chemical properties. The fluoride ion’s high electronegativity and small size make it a potent nucleophile, enhancing the compound’s reactivity in nucleophilic substitution reactions. Additionally, its ability to target mitochondria makes it valuable in biological and medical research.
Biological Activity
Methyltriphenylphosphanium fluoride (MTPPF) is a quaternary ammonium compound that has garnered attention in the fields of medicinal chemistry and biochemistry due to its unique biological properties. This article delves into the biological activity of MTPPF, highlighting its mechanisms of action, potential therapeutic applications, and relevant research findings.
Chemical Structure and Properties
MTPPF is characterized by its triphenylphosphonium moiety, which enhances its lipophilicity and ability to penetrate biological membranes. This property is crucial for its biological activity, particularly in targeting mitochondria, where it can influence various cellular processes.
Mechanisms of Biological Activity
- Cell Membrane Interaction : MTPPF exhibits significant interaction with cellular membranes due to its lipophilic nature. This allows it to cross lipid bilayers effectively, making it a candidate for mitochondrial targeting in therapeutic applications .
- Reactive Oxygen Species (ROS) Generation : The compound has been shown to induce oxidative stress through the generation of ROS. This mechanism is linked to its potential cytotoxic effects, particularly in cancer cells .
- Inhibition of Tumor Growth : Research indicates that MTPPF can inhibit angiogenesis and exhibit selective cytotoxicity against various cancer cell lines, including CEM (human leukemia), H460 (lung cancer), and HT-29 (colon cancer). The IC50 values for these effects are notably low, suggesting potent anti-cancer properties .
- Mechanistic Insights : Studies suggest that MTPPF may exert its effects through the inhibition of tubulin polymerization, which is vital for cell division and growth. By binding to the colchicine-binding site on β-tubulin, MTPPF disrupts microtubule formation, thereby hindering cancer cell proliferation .
Case Studies and Experimental Evidence
- Cytotoxicity Assays : In vitro studies have demonstrated that MTPPF shows selective toxicity towards cancer cells while sparing normal cells like VERO cells. For instance, one study reported an IC50 value of 0.1 μM against CEM cells, indicating high potency .
- Animal Models : Preclinical studies involving animal models have corroborated the cytotoxic effects observed in vitro. These studies often measure tumor size reduction and survival rates following treatment with MTPPF .
- Mitochondrial Targeting : The ability of MTPPF to accumulate in mitochondria has been linked to its role in inducing apoptosis in cancer cells. This mitochondrial localization is critical for enhancing the compound's therapeutic efficacy against tumors .
Data Summary
The following table summarizes key research findings related to MTPPF:
Properties
Molecular Formula |
C19H18FP |
---|---|
Molecular Weight |
296.3 g/mol |
IUPAC Name |
methyl(triphenyl)phosphanium;fluoride |
InChI |
InChI=1S/C19H18P.FH/c1-20(17-11-5-2-6-12-17,18-13-7-3-8-14-18)19-15-9-4-10-16-19;/h2-16H,1H3;1H/q+1;/p-1 |
InChI Key |
AIKVJULUSXQNMQ-UHFFFAOYSA-M |
Canonical SMILES |
C[P+](C1=CC=CC=C1)(C2=CC=CC=C2)C3=CC=CC=C3.[F-] |
Origin of Product |
United States |
Disclaimer and Information on In-Vitro Research Products
Please be aware that all articles and product information presented on BenchChem are intended solely for informational purposes. The products available for purchase on BenchChem are specifically designed for in-vitro studies, which are conducted outside of living organisms. In-vitro studies, derived from the Latin term "in glass," involve experiments performed in controlled laboratory settings using cells or tissues. It is important to note that these products are not categorized as medicines or drugs, and they have not received approval from the FDA for the prevention, treatment, or cure of any medical condition, ailment, or disease. We must emphasize that any form of bodily introduction of these products into humans or animals is strictly prohibited by law. It is essential to adhere to these guidelines to ensure compliance with legal and ethical standards in research and experimentation.