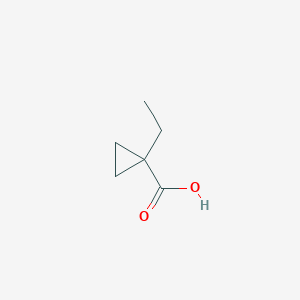
1-Ethylcyclopropane-1-carboxylic acid
Overview
Description
1-Ethylcyclopropane-1-carboxylic acid is an organic compound featuring a cyclopropane ring substituted with an ethyl group and a carboxylic acid group
Preparation Methods
Synthetic Routes and Reaction Conditions: 1-Ethylcyclopropane-1-carboxylic acid can be synthesized through several methods. One common approach involves the cyclopropanation of alkenes using carbenes or carbenoids. For instance, the reaction of ethyl diazoacetate with an alkene in the presence of a transition metal catalyst can yield the desired cyclopropane derivative . Another method involves the intramolecular cyclization of γ-substituted amino acid derivatives .
Industrial Production Methods: Industrial production of this compound typically involves large-scale cyclopropanation reactions using optimized catalysts and reaction conditions to ensure high yield and purity. The use of continuous flow reactors and advanced separation techniques can further enhance the efficiency of the production process .
Chemical Reactions Analysis
Types of Reactions: 1-Ethylcyclopropane-1-carboxylic acid undergoes various chemical reactions, including:
Oxidation: The carboxylic acid group can be oxidized to form corresponding derivatives.
Reduction: Reduction reactions can convert the carboxylic acid group to an alcohol or other functional groups.
Substitution: The ethyl group or the cyclopropane ring can undergo substitution reactions with suitable reagents.
Common Reagents and Conditions:
Oxidation: Common oxidizing agents include potassium permanganate and chromium trioxide.
Reduction: Reducing agents such as lithium aluminum hydride or sodium borohydride are often used.
Substitution: Halogenating agents like bromine or chlorine can be used for substitution reactions.
Major Products: The major products formed from these reactions depend on the specific reagents and conditions used. For example, oxidation of the carboxylic acid group can yield ketones or aldehydes, while reduction can produce alcohols .
Scientific Research Applications
1-Ethylcyclopropane-1-carboxylic acid has several applications in scientific research:
Chemistry: It serves as a building block for the synthesis of more complex organic molecules.
Biology: It is used in studies related to enzyme inhibition and metabolic pathways.
Industry: It is used in the production of specialty chemicals and materials.
Mechanism of Action
The mechanism by which 1-ethylcyclopropane-1-carboxylic acid exerts its effects involves interactions with specific molecular targets and pathways. For instance, in biological systems, it may act as an inhibitor of certain enzymes or as a precursor in metabolic pathways. The cyclopropane ring’s strained nature makes it highly reactive, allowing it to participate in various chemical transformations .
Comparison with Similar Compounds
Cyclopropane: A simple cycloalkane with a three-membered ring.
Cyclobutane: A four-membered ring cycloalkane.
Cyclopentane: A five-membered ring cycloalkane.
Uniqueness: 1-Ethylcyclopropane-1-carboxylic acid is unique due to the presence of both an ethyl group and a carboxylic acid group on the cyclopropane ring. This combination imparts distinct chemical and physical properties, making it valuable for specific applications in research and industry .
Properties
IUPAC Name |
1-ethylcyclopropane-1-carboxylic acid | |
---|---|---|
Source | PubChem | |
URL | https://pubchem.ncbi.nlm.nih.gov | |
Description | Data deposited in or computed by PubChem | |
InChI |
InChI=1S/C6H10O2/c1-2-6(3-4-6)5(7)8/h2-4H2,1H3,(H,7,8) | |
Source | PubChem | |
URL | https://pubchem.ncbi.nlm.nih.gov | |
Description | Data deposited in or computed by PubChem | |
InChI Key |
POZHVHBTFKOUEN-UHFFFAOYSA-N | |
Source | PubChem | |
URL | https://pubchem.ncbi.nlm.nih.gov | |
Description | Data deposited in or computed by PubChem | |
Canonical SMILES |
CCC1(CC1)C(=O)O | |
Source | PubChem | |
URL | https://pubchem.ncbi.nlm.nih.gov | |
Description | Data deposited in or computed by PubChem | |
Molecular Formula |
C6H10O2 | |
Source | PubChem | |
URL | https://pubchem.ncbi.nlm.nih.gov | |
Description | Data deposited in or computed by PubChem | |
DSSTOX Substance ID |
DTXSID10620839 | |
Record name | 1-Ethylcyclopropane-1-carboxylic acid | |
Source | EPA DSSTox | |
URL | https://comptox.epa.gov/dashboard/DTXSID10620839 | |
Description | DSSTox provides a high quality public chemistry resource for supporting improved predictive toxicology. | |
Molecular Weight |
114.14 g/mol | |
Source | PubChem | |
URL | https://pubchem.ncbi.nlm.nih.gov | |
Description | Data deposited in or computed by PubChem | |
CAS No. |
150864-95-2 | |
Record name | 1-Ethylcyclopropane-1-carboxylic acid | |
Source | EPA DSSTox | |
URL | https://comptox.epa.gov/dashboard/DTXSID10620839 | |
Description | DSSTox provides a high quality public chemistry resource for supporting improved predictive toxicology. | |
Record name | 1-ethylcyclopropane-1-carboxylic acid | |
Source | European Chemicals Agency (ECHA) | |
URL | https://echa.europa.eu/information-on-chemicals | |
Description | The European Chemicals Agency (ECHA) is an agency of the European Union which is the driving force among regulatory authorities in implementing the EU's groundbreaking chemicals legislation for the benefit of human health and the environment as well as for innovation and competitiveness. | |
Explanation | Use of the information, documents and data from the ECHA website is subject to the terms and conditions of this Legal Notice, and subject to other binding limitations provided for under applicable law, the information, documents and data made available on the ECHA website may be reproduced, distributed and/or used, totally or in part, for non-commercial purposes provided that ECHA is acknowledged as the source: "Source: European Chemicals Agency, http://echa.europa.eu/". Such acknowledgement must be included in each copy of the material. ECHA permits and encourages organisations and individuals to create links to the ECHA website under the following cumulative conditions: Links can only be made to webpages that provide a link to the Legal Notice page. | |
Synthesis routes and methods
Procedure details
Retrosynthesis Analysis
AI-Powered Synthesis Planning: Our tool employs the Template_relevance Pistachio, Template_relevance Bkms_metabolic, Template_relevance Pistachio_ringbreaker, Template_relevance Reaxys, Template_relevance Reaxys_biocatalysis model, leveraging a vast database of chemical reactions to predict feasible synthetic routes.
One-Step Synthesis Focus: Specifically designed for one-step synthesis, it provides concise and direct routes for your target compounds, streamlining the synthesis process.
Accurate Predictions: Utilizing the extensive PISTACHIO, BKMS_METABOLIC, PISTACHIO_RINGBREAKER, REAXYS, REAXYS_BIOCATALYSIS database, our tool offers high-accuracy predictions, reflecting the latest in chemical research and data.
Strategy Settings
Precursor scoring | Relevance Heuristic |
---|---|
Min. plausibility | 0.01 |
Model | Template_relevance |
Template Set | Pistachio/Bkms_metabolic/Pistachio_ringbreaker/Reaxys/Reaxys_biocatalysis |
Top-N result to add to graph | 6 |
Feasible Synthetic Routes
Disclaimer and Information on In-Vitro Research Products
Please be aware that all articles and product information presented on BenchChem are intended solely for informational purposes. The products available for purchase on BenchChem are specifically designed for in-vitro studies, which are conducted outside of living organisms. In-vitro studies, derived from the Latin term "in glass," involve experiments performed in controlled laboratory settings using cells or tissues. It is important to note that these products are not categorized as medicines or drugs, and they have not received approval from the FDA for the prevention, treatment, or cure of any medical condition, ailment, or disease. We must emphasize that any form of bodily introduction of these products into humans or animals is strictly prohibited by law. It is essential to adhere to these guidelines to ensure compliance with legal and ethical standards in research and experimentation.