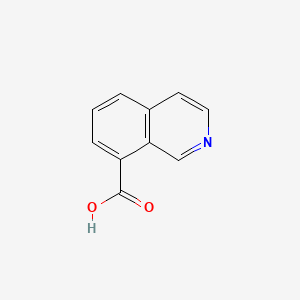
Isoquinoline-8-carboxylic acid
Overview
Description
Isoquinoline-8-carboxylic acid is an organic compound with the empirical formula C10H7NO2 . It is a solid substance and is part of a collection of unique chemicals provided by Sigma-Aldrich .
Synthesis Analysis
The synthesis of Isoquinoline and its derivatives has been a topic of interest in organic and medicinal chemistry . Therefore, the development of new efficient processes for isoquinoline and its derivatives that are characterized by easier operation, milder conditions, and higher yields has been stimulated .Molecular Structure Analysis
The molecular structure of Isoquinoline-8-carboxylic acid consists of a benzene ring fused with a pyridine ring . The InChI key for this compound is VMNZQPRIUSJCOH-UHFFFAOYSA-N .Physical And Chemical Properties Analysis
Isoquinoline-8-carboxylic acid is a solid substance . It has a molecular weight of 173.17 . The compound’s melting point is between 263°C to 267°C .Scientific Research Applications
Synthesis of Isoquinoline Alkaloids
Isoquinoline alkaloids are a prominent class of compounds with a wide range of biological activities. The synthesis of isoquinoline and its derivatives, including IQ8CA, is a critical area of research. IQ8CA serves as a precursor in the synthesis of complex isoquinoline alkaloids, which are used in anti-cancer and anti-malarial drugs .
Antiviral Agent Development
IQ8CA and its derivatives have shown promise as antiviral agents. They interfere with multiple pathways crucial for viral replication, such as nuclear factor-κB and mitogen-activated protein kinase/extracellular-signal-regulated kinase pathways. This makes IQ8CA a valuable compound in the study of new antiviral drugs, especially in the context of emerging diseases like COVID-19 .
Antibacterial Applications
The antibacterial activity of IQ8CA derivatives has been explored, with some compounds exhibiting high activity against plant bacteria. This suggests potential for IQ8CA in developing innovative, sustainable bactericides for agricultural use .
Scanning Electron Microscopy (SEM)
IQ8CA has been used in SEM studies to evaluate the ultrastructure of bacterial cells. This application is crucial for understanding the mode of action of antibacterial compounds at a microscopic level .
Catalyst-free Synthesis Processes
IQ8CA has been involved in catalyst-free synthesis processes in water. This approach is environmentally friendly and offers a sustainable method for synthesizing heterocyclic compounds, which are essential in pharmaceutical chemistry .
Drug Discovery and SAR Analysis
IQ8CA plays a role in the drug discovery process, particularly in structure-activity relationship (SAR) analysis. Its structure allows for the exploration of different modifications that can enhance biological activity, leading to the development of new therapeutic agents .
Safety And Hazards
Future Directions
properties
IUPAC Name |
isoquinoline-8-carboxylic acid | |
---|---|---|
Source | PubChem | |
URL | https://pubchem.ncbi.nlm.nih.gov | |
Description | Data deposited in or computed by PubChem | |
InChI |
InChI=1S/C10H7NO2/c12-10(13)8-3-1-2-7-4-5-11-6-9(7)8/h1-6H,(H,12,13) | |
Source | PubChem | |
URL | https://pubchem.ncbi.nlm.nih.gov | |
Description | Data deposited in or computed by PubChem | |
InChI Key |
VMNZQPRIUSJCOH-UHFFFAOYSA-N | |
Source | PubChem | |
URL | https://pubchem.ncbi.nlm.nih.gov | |
Description | Data deposited in or computed by PubChem | |
Canonical SMILES |
C1=CC2=C(C=NC=C2)C(=C1)C(=O)O | |
Source | PubChem | |
URL | https://pubchem.ncbi.nlm.nih.gov | |
Description | Data deposited in or computed by PubChem | |
Molecular Formula |
C10H7NO2 | |
Source | PubChem | |
URL | https://pubchem.ncbi.nlm.nih.gov | |
Description | Data deposited in or computed by PubChem | |
DSSTOX Substance ID |
DTXSID40612929 | |
Record name | Isoquinoline-8-carboxylic acid | |
Source | EPA DSSTox | |
URL | https://comptox.epa.gov/dashboard/DTXSID40612929 | |
Description | DSSTox provides a high quality public chemistry resource for supporting improved predictive toxicology. | |
Molecular Weight |
173.17 g/mol | |
Source | PubChem | |
URL | https://pubchem.ncbi.nlm.nih.gov | |
Description | Data deposited in or computed by PubChem | |
Product Name |
Isoquinoline-8-carboxylic acid | |
CAS RN |
61563-43-7 | |
Record name | Isoquinoline-8-carboxylic acid | |
Source | EPA DSSTox | |
URL | https://comptox.epa.gov/dashboard/DTXSID40612929 | |
Description | DSSTox provides a high quality public chemistry resource for supporting improved predictive toxicology. | |
Record name | Isoquinoline-8-carboxylic acid | |
Source | European Chemicals Agency (ECHA) | |
URL | https://echa.europa.eu/information-on-chemicals | |
Description | The European Chemicals Agency (ECHA) is an agency of the European Union which is the driving force among regulatory authorities in implementing the EU's groundbreaking chemicals legislation for the benefit of human health and the environment as well as for innovation and competitiveness. | |
Explanation | Use of the information, documents and data from the ECHA website is subject to the terms and conditions of this Legal Notice, and subject to other binding limitations provided for under applicable law, the information, documents and data made available on the ECHA website may be reproduced, distributed and/or used, totally or in part, for non-commercial purposes provided that ECHA is acknowledged as the source: "Source: European Chemicals Agency, http://echa.europa.eu/". Such acknowledgement must be included in each copy of the material. ECHA permits and encourages organisations and individuals to create links to the ECHA website under the following cumulative conditions: Links can only be made to webpages that provide a link to the Legal Notice page. | |
Retrosynthesis Analysis
AI-Powered Synthesis Planning: Our tool employs the Template_relevance Pistachio, Template_relevance Bkms_metabolic, Template_relevance Pistachio_ringbreaker, Template_relevance Reaxys, Template_relevance Reaxys_biocatalysis model, leveraging a vast database of chemical reactions to predict feasible synthetic routes.
One-Step Synthesis Focus: Specifically designed for one-step synthesis, it provides concise and direct routes for your target compounds, streamlining the synthesis process.
Accurate Predictions: Utilizing the extensive PISTACHIO, BKMS_METABOLIC, PISTACHIO_RINGBREAKER, REAXYS, REAXYS_BIOCATALYSIS database, our tool offers high-accuracy predictions, reflecting the latest in chemical research and data.
Strategy Settings
Precursor scoring | Relevance Heuristic |
---|---|
Min. plausibility | 0.01 |
Model | Template_relevance |
Template Set | Pistachio/Bkms_metabolic/Pistachio_ringbreaker/Reaxys/Reaxys_biocatalysis |
Top-N result to add to graph | 6 |
Feasible Synthetic Routes
Disclaimer and Information on In-Vitro Research Products
Please be aware that all articles and product information presented on BenchChem are intended solely for informational purposes. The products available for purchase on BenchChem are specifically designed for in-vitro studies, which are conducted outside of living organisms. In-vitro studies, derived from the Latin term "in glass," involve experiments performed in controlled laboratory settings using cells or tissues. It is important to note that these products are not categorized as medicines or drugs, and they have not received approval from the FDA for the prevention, treatment, or cure of any medical condition, ailment, or disease. We must emphasize that any form of bodily introduction of these products into humans or animals is strictly prohibited by law. It is essential to adhere to these guidelines to ensure compliance with legal and ethical standards in research and experimentation.