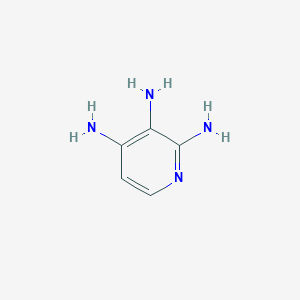
Pyridine-2,3,4-triamine
Overview
Description
Pyridine-2,3,4-triamine is an organic compound with the molecular formula C5H8N4 It is a derivative of pyridine, a six-membered aromatic ring containing one nitrogen atom this compound is characterized by the presence of three amino groups (-NH2) attached to the 2nd, 3rd, and 4th positions of the pyridine ring
Mechanism of Action
Target of Action
Pyridine-2,3,4-triamine is a nitrogen-containing heterocyclic compound . Similar compounds have shown a wide range of biological activities, such as antimicrobial, anti-inflammatory, antiviral, antifungal, antioxidant, antitumor, and kinase inhibitory .
Mode of Action
It is known that nitrogen-containing heterocycles like this compound are employed in different applications such as pharmaceuticals, organic materials, natural products, and mainly in bioactive molecules .
Biochemical Pathways
Nitrogen-containing heterocycles are precursors to the nicotinamide-derived redox cofactor, nicotinamide adenine dinucleotide (nad), its reduced form nadh, its phosphate parent nadp, and the reduced form nadph, collectively referred to as nad(p)(h) . These compounds play a key role in various biochemical pathways.
Result of Action
Similar compounds have shown a wide range of biological activities, such as antimicrobial, anti-inflammatory, antiviral, antifungal, antioxidant, antitumor, and kinase inhibitory .
Action Environment
It is known that environmental factors can significantly influence the action of similar compounds .
Biochemical Analysis
Biochemical Properties
Pyridine-2,3,4-triamine plays a significant role in biochemical reactions, particularly in the context of enzyme interactions. It has been observed to interact with various enzymes, including those involved in oxidation-reduction processes. For instance, this compound can act as a substrate for certain oxidoreductases, facilitating electron transfer reactions. Additionally, it may interact with proteins that contain pyridine nucleotide cofactors, such as NAD+ and NADP+, influencing their activity and stability .
Cellular Effects
This compound has notable effects on cellular processes. It can influence cell signaling pathways by modulating the activity of key signaling molecules. For example, this compound may affect the phosphorylation status of proteins involved in signal transduction, thereby altering gene expression and cellular metabolism. Studies have shown that this compound can impact the expression of genes related to oxidative stress response and metabolic regulation .
Molecular Mechanism
The molecular mechanism of action of this compound involves its binding interactions with biomolecules. It can bind to specific sites on enzymes, leading to either inhibition or activation of their catalytic activity. For instance, this compound may inhibit the activity of certain kinases by competing with ATP for binding sites. Additionally, it can induce conformational changes in proteins, affecting their function and stability. These interactions can result in changes in gene expression and cellular responses .
Temporal Effects in Laboratory Settings
In laboratory settings, the effects of this compound can vary over time. The compound’s stability and degradation are critical factors that influence its long-term effects on cellular function. This compound is relatively stable under standard laboratory conditions, but it may undergo degradation when exposed to extreme pH or temperature. Long-term studies have shown that this compound can have sustained effects on cellular metabolism and gene expression, with some effects persisting even after the compound is no longer present .
Dosage Effects in Animal Models
The effects of this compound in animal models are dose-dependent. At low doses, the compound may exhibit beneficial effects, such as enhancing metabolic activity and reducing oxidative stress. At high doses, this compound can be toxic, leading to adverse effects such as cellular damage and organ dysfunction. Studies have identified threshold doses beyond which the compound’s toxicity becomes apparent, highlighting the importance of careful dosage regulation in experimental settings .
Metabolic Pathways
This compound is involved in several metabolic pathways, including those related to pyridine nucleotide metabolism. It can be metabolized by enzymes such as pyridine nucleotide transhydrogenase, which facilitates the transfer of electrons between NADH and NADPH. Additionally, this compound can influence the levels of metabolites involved in redox reactions, thereby affecting overall metabolic flux and cellular energy balance .
Transport and Distribution
Within cells and tissues, this compound is transported and distributed through specific transporters and binding proteins. These transporters facilitate the uptake and efflux of the compound, ensuring its proper localization within cellular compartments. This compound can accumulate in certain tissues, where it may exert localized effects on cellular function and metabolism .
Subcellular Localization
The subcellular localization of this compound is influenced by targeting signals and post-translational modifications. The compound can be directed to specific organelles, such as mitochondria or the endoplasmic reticulum, where it may interact with organelle-specific enzymes and proteins. These interactions can modulate the activity and function of the compound, contributing to its overall biochemical effects .
Preparation Methods
Synthetic Routes and Reaction Conditions: Pyridine-2,3,4-triamine can be synthesized through several methods. One common approach involves the nucleophilic aromatic substitution of a halogenated pyridine derivative with ammonia or an amine. For example, 2,3,4-trichloropyridine can be reacted with ammonia under high pressure and temperature to yield this compound .
Industrial Production Methods: Industrial production of this compound typically involves large-scale synthesis using optimized reaction conditions to maximize yield and purity. The process may include steps such as purification through recrystallization or distillation to remove impurities and obtain the desired product in high purity.
Chemical Reactions Analysis
Types of Reactions: Pyridine-2,3,4-triamine undergoes various chemical reactions, including:
Oxidation: It can be oxidized to form corresponding nitroso or nitro derivatives.
Reduction: Reduction reactions can convert it into different amine derivatives.
Common Reagents and Conditions:
Oxidation: Common oxidizing agents include hydrogen peroxide and potassium permanganate.
Reduction: Reducing agents such as lithium aluminum hydride or sodium borohydride are used.
Substitution: Electrophiles like alkyl halides or acyl chlorides are commonly used in substitution reactions.
Major Products Formed: The major products formed from these reactions depend on the specific reagents and conditions used. For example, oxidation may yield nitroso or nitro derivatives, while substitution reactions can produce a variety of substituted pyridine compounds.
Scientific Research Applications
Pyridine-2,3,4-triamine has several scientific research applications, including:
Chemistry: It is used as a building block in the synthesis of more complex organic molecules and heterocyclic compounds.
Biology: It serves as a precursor for the synthesis of biologically active molecules, including potential pharmaceuticals.
Medicine: Research is ongoing to explore its potential as a therapeutic agent due to its ability to interact with biological targets.
Industry: It is used in the production of polymers, dyes, and other industrial chemicals.
Comparison with Similar Compounds
Pyridine-2,3,5-triamine: Similar structure but with the amino group at the 5th position instead of the 4th.
Pyridine-2,4,6-triamine: Contains amino groups at the 2nd, 4th, and 6th positions.
Pyridine-3,4,5-triamine: Amino groups are located at the 3rd, 4th, and 5th positions.
Uniqueness: Pyridine-2,3,4-triamine is unique due to the specific arrangement of its amino groups, which can influence its reactivity and interactions with other molecules. This unique arrangement can lead to different chemical and biological properties compared to its isomers and other similar compounds .
Properties
IUPAC Name |
pyridine-2,3,4-triamine | |
---|---|---|
Source | PubChem | |
URL | https://pubchem.ncbi.nlm.nih.gov | |
Description | Data deposited in or computed by PubChem | |
InChI |
InChI=1S/C5H8N4/c6-3-1-2-9-5(8)4(3)7/h1-2H,7H2,(H4,6,8,9) | |
Source | PubChem | |
URL | https://pubchem.ncbi.nlm.nih.gov | |
Description | Data deposited in or computed by PubChem | |
InChI Key |
APXBXAJWVZTKSE-UHFFFAOYSA-N | |
Source | PubChem | |
URL | https://pubchem.ncbi.nlm.nih.gov | |
Description | Data deposited in or computed by PubChem | |
Canonical SMILES |
C1=CN=C(C(=C1N)N)N | |
Source | PubChem | |
URL | https://pubchem.ncbi.nlm.nih.gov | |
Description | Data deposited in or computed by PubChem | |
Molecular Formula |
C5H8N4 | |
Source | PubChem | |
URL | https://pubchem.ncbi.nlm.nih.gov | |
Description | Data deposited in or computed by PubChem | |
DSSTOX Substance ID |
DTXSID90622735 | |
Record name | Pyridine-2,3,4-triamine | |
Source | EPA DSSTox | |
URL | https://comptox.epa.gov/dashboard/DTXSID90622735 | |
Description | DSSTox provides a high quality public chemistry resource for supporting improved predictive toxicology. | |
Molecular Weight |
124.14 g/mol | |
Source | PubChem | |
URL | https://pubchem.ncbi.nlm.nih.gov | |
Description | Data deposited in or computed by PubChem | |
CAS No. |
52559-11-2 | |
Record name | Pyridine-2,3,4-triamine | |
Source | EPA DSSTox | |
URL | https://comptox.epa.gov/dashboard/DTXSID90622735 | |
Description | DSSTox provides a high quality public chemistry resource for supporting improved predictive toxicology. | |
Retrosynthesis Analysis
AI-Powered Synthesis Planning: Our tool employs the Template_relevance Pistachio, Template_relevance Bkms_metabolic, Template_relevance Pistachio_ringbreaker, Template_relevance Reaxys, Template_relevance Reaxys_biocatalysis model, leveraging a vast database of chemical reactions to predict feasible synthetic routes.
One-Step Synthesis Focus: Specifically designed for one-step synthesis, it provides concise and direct routes for your target compounds, streamlining the synthesis process.
Accurate Predictions: Utilizing the extensive PISTACHIO, BKMS_METABOLIC, PISTACHIO_RINGBREAKER, REAXYS, REAXYS_BIOCATALYSIS database, our tool offers high-accuracy predictions, reflecting the latest in chemical research and data.
Strategy Settings
Precursor scoring | Relevance Heuristic |
---|---|
Min. plausibility | 0.01 |
Model | Template_relevance |
Template Set | Pistachio/Bkms_metabolic/Pistachio_ringbreaker/Reaxys/Reaxys_biocatalysis |
Top-N result to add to graph | 6 |
Feasible Synthetic Routes
Disclaimer and Information on In-Vitro Research Products
Please be aware that all articles and product information presented on BenchChem are intended solely for informational purposes. The products available for purchase on BenchChem are specifically designed for in-vitro studies, which are conducted outside of living organisms. In-vitro studies, derived from the Latin term "in glass," involve experiments performed in controlled laboratory settings using cells or tissues. It is important to note that these products are not categorized as medicines or drugs, and they have not received approval from the FDA for the prevention, treatment, or cure of any medical condition, ailment, or disease. We must emphasize that any form of bodily introduction of these products into humans or animals is strictly prohibited by law. It is essential to adhere to these guidelines to ensure compliance with legal and ethical standards in research and experimentation.