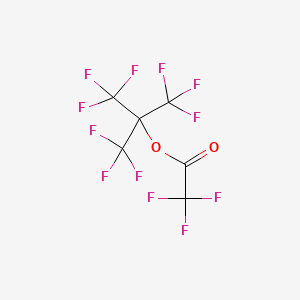
Perfluoro-t-butyl trifluoroacetate
- Click on QUICK INQUIRY to receive a quote from our team of experts.
- With the quality product at a COMPETITIVE price, you can focus more on your research.
Overview
Description
Perfluoro-t-butyl trifluoroacetate is a chemical compound with the molecular formula C6F12O2 . It is used in the method of producing elastic fluorohydrocarbon resin .
Synthesis Analysis
The selective introduction of perfluoro-tert-butyl group (PFtB, the bulkier analogue of CF3 group) into arenes has long been sought after but remains a formidable task . A general synthetic protocol to realize aromatic perfluoro-tert-butylation has been reported. The key to the success is the identification of PFtB phenyl sulfone as a new source of PFtB anion, which reacts with arynes in a highly regioselective manner to afford perfluoro-tert-butylated arenes in high yields .Molecular Structure Analysis
The molecular structure of Perfluoro-t-butyl trifluoroacetate consists of 6 Carbon atoms, 2 Oxygen atoms, and 12 Fluorine atoms . The exact mass is 331.9706672 g/mol .Chemical Reactions Analysis
Perfluoro-tert-butyl trifluoroacetate can serve as a robust nucleophilic perfluoro-tert-butylating agent for various electrophiles . It reacts with arynes in a highly regioselective manner to afford perfluoro-tert-butylated arenes in high yields .Physical And Chemical Properties Analysis
Perfluoro-t-butyl trifluoroacetate has a molecular weight of 332.04 g/mol . It has a topological polar surface area of 26.3 Ų .Scientific Research Applications
Amphiphilic Polymer Synthesis for Drug Delivery
Perfluoro-t-butyl trifluoroacetate is utilized in the synthesis of semifluorinated amphiphilic polymers. These polymers have a hydrophilic head group and a fluorophilic tail, making them suitable for forming stable micelles in aqueous solutions . These micelles can be used for the delivery of hydrophobic drugs, providing a potential platform for theranostic applications where both therapy and diagnostic functions are combined .
Fluorinated Surfactants for Theranostic Nanoparticles
The compound’s derivatives serve as fluorinated surfactants in the design of theranostic nanoparticles. These nanoparticles can simultaneously carry therapeutic agents and provide imaging contrast for fluorine magnetic resonance imaging (F-MRI), aiding in the visualization of drug delivery and release processes .
Environmental Impact and Toxicity Analysis
Perfluoro-t-butyl trifluoroacetate is related to trifluoroacetic acid (TFA), a known environmental pollutant. Research into the toxicity, sources, and sinks of TFA provides insights into the broader implications of perfluoro compounds in the environment. This includes understanding the degradation products of various fluorocarbons and their impact on ecosystems .
Phase Transition Studies in Material Science
The thermal behavior and phase transitions of perfluoro-t-butyl trifluoroacetate derivatives, such as perfluoro-tert-butanol, are of interest in material science. Studying these properties can lead to the development of materials with specific thermal and phase transition characteristics for industrial applications .
Future Directions
Perfluoroaromatic reagents, such as Perfluoro-t-butyl trifluoroacetate, have found applications as protecting groups or activating groups in peptide synthesis and as orthogonal handles for peptide modification . They have applications in chemoselective ‘tagging’, stapling and bioconjugation of peptides and proteins, as well as tuning of ‘drug-like’ properties . This suggests a promising future for these reagents in biological chemistry .
Mechanism of Action
Target of Action
Perfluoro-t-butyl trifluoroacetate is a perfluorinated chemical compound . It is a component of the empirical therapeutic oxygen carrier called Oxycyte . Its primary targets are tissues that require oxygenation .
Mode of Action
Perfluoro-t-butyl trifluoroacetate, as a part of Oxycyte, is designed to enhance oxygen delivery to damaged tissues . It can carry oxygen with up to 5 times the efficiency of hemoglobin when used as an intravenous emulsion . This makes it an effective means of transporting oxygen to tissues and carrying carbon dioxide to the lungs for disposal .
Biochemical Pathways
It is known that fluorocarbons, like this compound, have strong gas-dissolving properties . When used with oxygen, they serve a dual role of healing the tissue as well as imaging .
Result of Action
The primary result of the action of Perfluoro-t-butyl trifluoroacetate is enhanced oxygen delivery to tissues . This can be particularly beneficial in conditions where oxygen supply is compromised, such as in ischemic stroke .
Action Environment
Environmental factors can influence the action, efficacy, and stability of Perfluoro-t-butyl trifluoroacetate. For instance, the compound’s action may be influenced by the presence of other substances in the environment . Furthermore, the compound is known to be a persistent pollutant in the environment .
properties
IUPAC Name |
[1,1,1,3,3,3-hexafluoro-2-(trifluoromethyl)propan-2-yl] 2,2,2-trifluoroacetate |
Source
|
---|---|---|
Source | PubChem | |
URL | https://pubchem.ncbi.nlm.nih.gov | |
Description | Data deposited in or computed by PubChem | |
InChI |
InChI=1S/C6F12O2/c7-2(8,9)1(19)20-3(4(10,11)12,5(13,14)15)6(16,17)18 |
Source
|
Source | PubChem | |
URL | https://pubchem.ncbi.nlm.nih.gov | |
Description | Data deposited in or computed by PubChem | |
InChI Key |
FXSOHPXIFIETIO-UHFFFAOYSA-N |
Source
|
Source | PubChem | |
URL | https://pubchem.ncbi.nlm.nih.gov | |
Description | Data deposited in or computed by PubChem | |
Canonical SMILES |
C(=O)(C(F)(F)F)OC(C(F)(F)F)(C(F)(F)F)C(F)(F)F |
Source
|
Source | PubChem | |
URL | https://pubchem.ncbi.nlm.nih.gov | |
Description | Data deposited in or computed by PubChem | |
Molecular Formula |
C6F12O2 |
Source
|
Source | PubChem | |
URL | https://pubchem.ncbi.nlm.nih.gov | |
Description | Data deposited in or computed by PubChem | |
DSSTOX Substance ID |
DTXSID40614100 |
Source
|
Record name | 1,1,1,3,3,3-Hexafluoro-2-(trifluoromethyl)propan-2-yl trifluoroacetate | |
Source | EPA DSSTox | |
URL | https://comptox.epa.gov/dashboard/DTXSID40614100 | |
Description | DSSTox provides a high quality public chemistry resource for supporting improved predictive toxicology. | |
Molecular Weight |
332.04 g/mol |
Source
|
Source | PubChem | |
URL | https://pubchem.ncbi.nlm.nih.gov | |
Description | Data deposited in or computed by PubChem | |
Product Name |
Perfluoro-t-butyl trifluoroacetate | |
CAS RN |
24165-10-4 |
Source
|
Record name | 1,1,1,3,3,3-Hexafluoro-2-(trifluoromethyl)propan-2-yl trifluoroacetate | |
Source | EPA DSSTox | |
URL | https://comptox.epa.gov/dashboard/DTXSID40614100 | |
Description | DSSTox provides a high quality public chemistry resource for supporting improved predictive toxicology. | |
Disclaimer and Information on In-Vitro Research Products
Please be aware that all articles and product information presented on BenchChem are intended solely for informational purposes. The products available for purchase on BenchChem are specifically designed for in-vitro studies, which are conducted outside of living organisms. In-vitro studies, derived from the Latin term "in glass," involve experiments performed in controlled laboratory settings using cells or tissues. It is important to note that these products are not categorized as medicines or drugs, and they have not received approval from the FDA for the prevention, treatment, or cure of any medical condition, ailment, or disease. We must emphasize that any form of bodily introduction of these products into humans or animals is strictly prohibited by law. It is essential to adhere to these guidelines to ensure compliance with legal and ethical standards in research and experimentation.