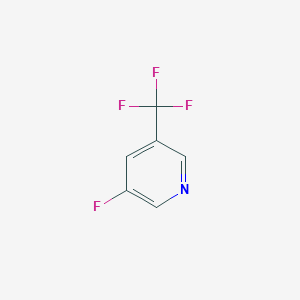
3-Fluoro-5-(trifluoromethyl)pyridine
Overview
Description
3-Fluoro-5-(trifluoromethyl)pyridine is a fluorinated pyridine derivative known for its unique chemical properties. The presence of both fluorine and trifluoromethyl groups in its structure imparts significant electron-withdrawing characteristics, making it a valuable compound in various chemical and industrial applications .
Mechanism of Action
Target of Action
3-Fluoro-5-(trifluoromethyl)pyridine (TFMP) and its derivatives are primarily used in the agrochemical and pharmaceutical industries . The biological activities of TFMP derivatives are thought to be due to the combination of the unique physicochemical properties of the fluorine atom and the unique characteristics of the pyridine moiety .
Mode of Action
It’s known that the biological activities of tfmp derivatives are thought to be due to the combination of the unique physicochemical properties of the fluorine atom and the unique characteristics of the pyridine moiety .
Biochemical Pathways
Tfmp and its derivatives have been used in the synthesis of several crop-protection products , suggesting that they may interact with biochemical pathways related to pest resistance.
Pharmacokinetics
The unique physicochemical properties of the fluorine atom and the pyridine moiety in tfmp derivatives may influence their pharmacokinetic properties .
Result of Action
Tfmp and its derivatives are primarily used in the agrochemical industry for the protection of crops from pests , suggesting that they may have pesticidal effects at the molecular and cellular levels.
Biochemical Analysis
Biochemical Properties
3-Fluoro-5-(trifluoromethyl)pyridine plays a crucial role in biochemical reactions, particularly in the synthesis of agrochemical and pharmaceutical compounds. This compound interacts with various enzymes and proteins, influencing their activity and stability. For instance, the trifluoromethyl group in this compound is known to enhance the binding affinity of the compound to target enzymes, thereby modulating their catalytic activity . Additionally, the fluorine atoms in the compound can form hydrogen bonds with amino acid residues in proteins, further stabilizing the enzyme-substrate complex .
Cellular Effects
The effects of this compound on cellular processes are profound. This compound has been shown to influence cell signaling pathways, gene expression, and cellular metabolism. In particular, this compound can modulate the activity of key signaling proteins, leading to alterations in downstream signaling cascades . This modulation can result in changes in gene expression patterns, affecting various cellular functions such as proliferation, differentiation, and apoptosis . Furthermore, the compound’s impact on cellular metabolism includes alterations in metabolic flux and metabolite levels, which can have significant implications for cell function and viability .
Molecular Mechanism
At the molecular level, this compound exerts its effects through several mechanisms. One of the primary mechanisms involves the binding of the compound to specific biomolecules, such as enzymes and receptors . This binding can lead to either inhibition or activation of the target biomolecule, depending on the nature of the interaction . For example, the trifluoromethyl group in this compound can act as an electron-withdrawing group, altering the electronic environment of the binding site and affecting the enzyme’s catalytic activity . Additionally, the compound can influence gene expression by interacting with transcription factors and other regulatory proteins, leading to changes in the transcriptional activity of target genes .
Temporal Effects in Laboratory Settings
The temporal effects of this compound in laboratory settings have been extensively studied. Over time, the stability and degradation of the compound can influence its biochemical activity . In vitro studies have shown that this compound remains stable under various experimental conditions, maintaining its activity over extended periods . In vivo studies have indicated that the compound can undergo metabolic degradation, leading to the formation of inactive metabolites . Long-term exposure to this compound has also been associated with changes in cellular function, including alterations in cell proliferation and differentiation .
Dosage Effects in Animal Models
The effects of this compound vary with different dosages in animal models. At low doses, the compound has been shown to exhibit beneficial effects, such as enhanced enzyme activity and improved cellular function . At high doses, this compound can induce toxic effects, including cellular damage and organ toxicity . Threshold effects have been observed, where the compound’s activity significantly changes at specific dosage levels . These findings highlight the importance of optimizing dosage to achieve the desired biochemical effects while minimizing adverse outcomes .
Metabolic Pathways
This compound is involved in several metabolic pathways, interacting with various enzymes and cofactors. The compound can be metabolized through oxidative and reductive pathways, leading to the formation of different metabolites . Enzymes such as cytochrome P450 play a crucial role in the metabolism of this compound, catalyzing its biotransformation into active or inactive forms . The compound’s effects on metabolic flux and metabolite levels can influence overall cellular metabolism, impacting various physiological processes .
Transport and Distribution
The transport and distribution of this compound within cells and tissues are mediated by specific transporters and binding proteins . The compound can be actively transported across cell membranes, accumulating in specific cellular compartments . Binding proteins can facilitate the distribution of this compound to target tissues, enhancing its bioavailability and efficacy . The localization and accumulation of the compound within cells can influence its biochemical activity and overall therapeutic potential .
Subcellular Localization
The subcellular localization of this compound is critical for its activity and function. The compound can be targeted to specific cellular compartments, such as the nucleus, mitochondria, or endoplasmic reticulum, through targeting signals or post-translational modifications . The localization of this compound within these compartments can affect its interactions with biomolecules and its overall biochemical activity . For example, the accumulation of the compound in the nucleus can influence gene expression by interacting with transcription factors and other nuclear proteins .
Preparation Methods
Synthetic Routes and Reaction Conditions
The synthesis of 3-Fluoro-5-(trifluoromethyl)pyridine typically involves the fluorination of pyridine derivatives. One common method includes the reaction of 2,3-dichloro-5-trichloromethylpyridine with anhydrous hydrogen fluoride at elevated temperatures (170°C to 200°C) and high pressure (at least 200 psig) in the absence of a catalyst . Another method involves the gas-phase chlorofluorination of β-picoline using chlorine and hydrogen fluoride in a fluidized-bed reactor, with chromium-based catalysts showing high activity .
Industrial Production Methods
Industrial production of this compound often employs continuous flow processes to ensure high yield and purity. The use of advanced catalytic systems and optimized reaction conditions allows for efficient large-scale synthesis .
Chemical Reactions Analysis
Types of Reactions
3-Fluoro-5-(trifluoromethyl)pyridine undergoes various chemical reactions, including:
Substitution Reactions: The compound can participate in nucleophilic substitution reactions due to the electron-withdrawing effects of the fluorine and trifluoromethyl groups.
Metalation Reactions: It can form pyridyllithium derivatives through metalation reactions, which are useful intermediates in further chemical transformations.
Common Reagents and Conditions
Common reagents used in reactions with this compound include organolithium reagents, halogenating agents, and various catalysts. Reaction conditions often involve low temperatures and inert atmospheres to prevent unwanted side reactions .
Major Products
Major products formed from reactions involving this compound include various substituted pyridine derivatives, which are valuable intermediates in the synthesis of pharmaceuticals and agrochemicals .
Scientific Research Applications
3-Fluoro-5-(trifluoromethyl)pyridine has a wide range of applications in scientific research:
Comparison with Similar Compounds
Similar Compounds
3-Chloro-2-fluoro-5-(trifluoromethyl)pyridine: Another halogenated pyridine derivative with similar electron-withdrawing properties.
2,3,5-Trichloropyridine: A chlorinated pyridine used in similar applications but with different reactivity due to the absence of fluorine.
Uniqueness
3-Fluoro-5-(trifluoromethyl)pyridine is unique due to the combined presence of fluorine and trifluoromethyl groups, which significantly enhance its chemical stability and reactivity. This makes it a versatile compound in various chemical syntheses and industrial applications .
Properties
IUPAC Name |
3-fluoro-5-(trifluoromethyl)pyridine | |
---|---|---|
Source | PubChem | |
URL | https://pubchem.ncbi.nlm.nih.gov | |
Description | Data deposited in or computed by PubChem | |
InChI |
InChI=1S/C6H3F4N/c7-5-1-4(2-11-3-5)6(8,9)10/h1-3H | |
Source | PubChem | |
URL | https://pubchem.ncbi.nlm.nih.gov | |
Description | Data deposited in or computed by PubChem | |
InChI Key |
GWTMPNXPVMDUTM-UHFFFAOYSA-N | |
Source | PubChem | |
URL | https://pubchem.ncbi.nlm.nih.gov | |
Description | Data deposited in or computed by PubChem | |
Canonical SMILES |
C1=C(C=NC=C1F)C(F)(F)F | |
Source | PubChem | |
URL | https://pubchem.ncbi.nlm.nih.gov | |
Description | Data deposited in or computed by PubChem | |
Molecular Formula |
C6H3F4N | |
Source | PubChem | |
URL | https://pubchem.ncbi.nlm.nih.gov | |
Description | Data deposited in or computed by PubChem | |
DSSTOX Substance ID |
DTXSID10601421 | |
Record name | 3-Fluoro-5-(trifluoromethyl)pyridine | |
Source | EPA DSSTox | |
URL | https://comptox.epa.gov/dashboard/DTXSID10601421 | |
Description | DSSTox provides a high quality public chemistry resource for supporting improved predictive toxicology. | |
Molecular Weight |
165.09 g/mol | |
Source | PubChem | |
URL | https://pubchem.ncbi.nlm.nih.gov | |
Description | Data deposited in or computed by PubChem | |
CAS No. |
1099597-96-2 | |
Record name | 3-Fluoro-5-(trifluoromethyl)pyridine | |
Source | CAS Common Chemistry | |
URL | https://commonchemistry.cas.org/detail?cas_rn=1099597-96-2 | |
Description | CAS Common Chemistry is an open community resource for accessing chemical information. Nearly 500,000 chemical substances from CAS REGISTRY cover areas of community interest, including common and frequently regulated chemicals, and those relevant to high school and undergraduate chemistry classes. This chemical information, curated by our expert scientists, is provided in alignment with our mission as a division of the American Chemical Society. | |
Explanation | The data from CAS Common Chemistry is provided under a CC-BY-NC 4.0 license, unless otherwise stated. | |
Record name | 3-Fluoro-5-(trifluoromethyl)pyridine | |
Source | EPA DSSTox | |
URL | https://comptox.epa.gov/dashboard/DTXSID10601421 | |
Description | DSSTox provides a high quality public chemistry resource for supporting improved predictive toxicology. | |
Retrosynthesis Analysis
AI-Powered Synthesis Planning: Our tool employs the Template_relevance Pistachio, Template_relevance Bkms_metabolic, Template_relevance Pistachio_ringbreaker, Template_relevance Reaxys, Template_relevance Reaxys_biocatalysis model, leveraging a vast database of chemical reactions to predict feasible synthetic routes.
One-Step Synthesis Focus: Specifically designed for one-step synthesis, it provides concise and direct routes for your target compounds, streamlining the synthesis process.
Accurate Predictions: Utilizing the extensive PISTACHIO, BKMS_METABOLIC, PISTACHIO_RINGBREAKER, REAXYS, REAXYS_BIOCATALYSIS database, our tool offers high-accuracy predictions, reflecting the latest in chemical research and data.
Strategy Settings
Precursor scoring | Relevance Heuristic |
---|---|
Min. plausibility | 0.01 |
Model | Template_relevance |
Template Set | Pistachio/Bkms_metabolic/Pistachio_ringbreaker/Reaxys/Reaxys_biocatalysis |
Top-N result to add to graph | 6 |
Feasible Synthetic Routes
Disclaimer and Information on In-Vitro Research Products
Please be aware that all articles and product information presented on BenchChem are intended solely for informational purposes. The products available for purchase on BenchChem are specifically designed for in-vitro studies, which are conducted outside of living organisms. In-vitro studies, derived from the Latin term "in glass," involve experiments performed in controlled laboratory settings using cells or tissues. It is important to note that these products are not categorized as medicines or drugs, and they have not received approval from the FDA for the prevention, treatment, or cure of any medical condition, ailment, or disease. We must emphasize that any form of bodily introduction of these products into humans or animals is strictly prohibited by law. It is essential to adhere to these guidelines to ensure compliance with legal and ethical standards in research and experimentation.