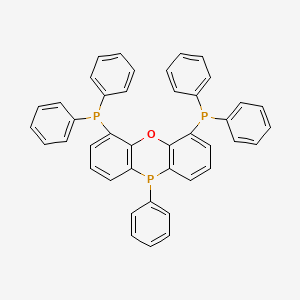
4,6-Bis(diphenylphosphino)-10-phenyl-10H-phenoxaphosphine
- Click on QUICK INQUIRY to receive a quote from our team of experts.
- With the quality product at a COMPETITIVE price, you can focus more on your research.
Overview
Description
4,6-Bis(diphenylphosphino)-10-phenyl-10H-phenoxaphosphinine is an organophosphorus compound known for its unique structure and versatile applications. This compound is characterized by the presence of two diphenylphosphino groups attached to a phenoxaphosphinine backbone. It is widely used as a ligand in coordination chemistry and catalysis due to its ability to form stable complexes with transition metals .
Preparation Methods
Synthetic Routes and Reaction Conditions
The synthesis of 4,6-Bis(diphenylphosphino)-10-phenyl-10H-phenoxaphosphinine typically involves the reaction of diphenylphosphine with a suitable phenoxaphosphinine precursor. One common method involves the reaction of 4,6-dichloro-10-phenyl-10H-phenoxaphosphinine with diphenylphosphine in the presence of a base such as sodium hydride. The reaction is usually carried out in an inert atmosphere to prevent oxidation .
Industrial Production Methods
Industrial production of this compound follows similar synthetic routes but on a larger scale. The reaction conditions are optimized to ensure high yield and purity. The use of continuous flow reactors and automated systems helps in scaling up the production while maintaining consistency and quality .
Chemical Reactions Analysis
Types of Reactions
4,6-Bis(diphenylphosphino)-10-phenyl-10H-phenoxaphosphinine undergoes various types of chemical reactions, including:
Oxidation: The compound can be oxidized to form phosphine oxides.
Reduction: Reduction reactions can convert the phosphine groups to phosphine hydrides.
Substitution: The phenyl groups can be substituted with other functional groups under appropriate conditions[][3].
Common Reagents and Conditions
Oxidation: Common oxidizing agents include hydrogen peroxide and oxygen.
Reduction: Reducing agents such as lithium aluminum hydride are used.
Substitution: Substitution reactions often require catalysts such as palladium or nickel complexes[][3].
Major Products Formed
Oxidation: Phosphine oxides.
Reduction: Phosphine hydrides.
Substitution: Various substituted derivatives depending on the reagents used[][3].
Scientific Research Applications
4,6-Bis(diphenylphosphino)-10-phenyl-10H-phenoxaphosphinine has a wide range of applications in scientific research:
Mechanism of Action
The mechanism of action of 4,6-Bis(diphenylphosphino)-10-phenyl-10H-phenoxaphosphinine primarily involves its ability to act as a ligand and form stable complexes with transition metals. The diphenylphosphino groups coordinate with metal ions, facilitating various catalytic processes. The phenoxaphosphinine backbone provides structural stability and enhances the compound’s reactivity. The molecular targets and pathways involved depend on the specific metal ion and the type of reaction being catalyzed .
Comparison with Similar Compounds
Similar Compounds
- 4,6-Bis(diphenylphosphino)-10,10-dimethylphenoxasilin (sixantphos)
- 4,5-Bis(diphenylphosphino)-9-isopropylidenexanthene (isopropxantphos)
- Bis-(2-diphenylphosphino)-p-tolyl ether (PTEphos)
Uniqueness
4,6-Bis(diphenylphosphino)-10-phenyl-10H-phenoxaphosphinine is unique due to its phenoxaphosphinine backbone, which provides enhanced stability and reactivity compared to other similar compounds. The presence of the phenyl group at the 10-position further distinguishes it from other ligands, offering unique electronic and steric properties that influence its coordination behavior and catalytic activity .
Properties
Molecular Formula |
C42H31OP3 |
---|---|
Molecular Weight |
644.6 g/mol |
IUPAC Name |
(6-diphenylphosphanyl-10-phenylphenoxaphosphinin-4-yl)-diphenylphosphane |
InChI |
InChI=1S/C42H31OP3/c1-6-18-32(19-7-1)44(33-20-8-2-9-21-33)37-28-16-30-39-41(37)43-42-38(29-17-31-40(42)46(39)36-26-14-5-15-27-36)45(34-22-10-3-11-23-34)35-24-12-4-13-25-35/h1-31H |
InChI Key |
NZHBREACEUUMIA-UHFFFAOYSA-N |
Canonical SMILES |
C1=CC=C(C=C1)P2C3=C(C(=CC=C3)P(C4=CC=CC=C4)C5=CC=CC=C5)OC6=C2C=CC=C6P(C7=CC=CC=C7)C8=CC=CC=C8 |
Origin of Product |
United States |
Disclaimer and Information on In-Vitro Research Products
Please be aware that all articles and product information presented on BenchChem are intended solely for informational purposes. The products available for purchase on BenchChem are specifically designed for in-vitro studies, which are conducted outside of living organisms. In-vitro studies, derived from the Latin term "in glass," involve experiments performed in controlled laboratory settings using cells or tissues. It is important to note that these products are not categorized as medicines or drugs, and they have not received approval from the FDA for the prevention, treatment, or cure of any medical condition, ailment, or disease. We must emphasize that any form of bodily introduction of these products into humans or animals is strictly prohibited by law. It is essential to adhere to these guidelines to ensure compliance with legal and ethical standards in research and experimentation.