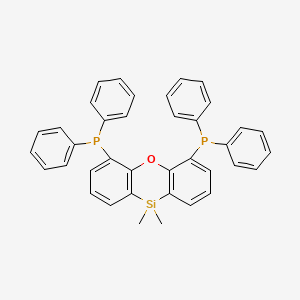
Sixantphos
- Click on QUICK INQUIRY to receive a quote from our team of experts.
- With the quality product at a COMPETITIVE price, you can focus more on your research.
Overview
Description
Sixantphos (4,6-bis(diphenylphosphino)-10,10-dimethylphenoxasilin) is a diphosphine ligand belonging to the xanthene-derived ligand family, characterized by a rigid heterocyclic backbone containing silicon (Si) and oxygen (O) atoms . Its structure comprises two diphenylphosphine groups attached to a phenoxasilin framework, which imparts unique steric and electronic properties. This ligand is widely employed in coordination chemistry and homogeneous catalysis, particularly in rhodium- and palladium-catalyzed reactions such as hydroformylation, isomerization, and carbonylation . Its conformational flexibility and tunable bite angle (the P–M–P angle) make it adaptable to diverse metal centers, enabling high selectivity and activity in catalytic transformations .
Preparation Methods
Multistep Organic Synthesis of the Xanthene Backbone
The initial phase involves constructing the xanthene core with appropriate substitution patterns to enable subsequent phosphination. The synthesis generally begins with 9,9-dimethyl-9H-xanthene, which is subjected to selective functionalization at positions 4 and 5.
Preparation of 4,5-Bis(hydroxymethyl)xanthene
- Reaction: Selective deprotonation of the xanthene backbone at activated positions 4 and 5 using n-butyllithium (n-BuLi) in the presence of tetramethylethylenediamine (TMEDA) under inert atmosphere.
- Conditions: Reflux at ambient temperature, followed by quenching with N,N-dimethyl-formamide (DMF) to introduce aldehyde groups.
- Outcome: High-yield formation (~90%) of 4,5-bis(aldehyde)xanthene, serving as a precursor for further functionalization.
Conversion to 4,5-Bis(bromomethyl)-xanthene
- Reaction: Bromination of the aldehyde groups using phosphorus tribromide (PBr₃).
- Conditions: Conducted in dry solvents such as dichloromethane (DCM) at low temperature to prevent overbromination.
- Outcome: Formation of 4,5-bis(bromomethyl)-xanthene with yields around 60-70%, facilitating subsequent phosphine attachment.
Crystallization and Purification
- Method: Recrystallization from solvents like methanol or ethanol ensures high purity.
- Yield: Overall yields from initial xanthene precursor to final ligand are approximately 50-70%, contingent on reaction efficiencies at each step.
Summary Table of Preparation Methods
Research Results and Characterization Data
- Spectroscopic confirmation:
- NMR: Characteristic signals for aromatic protons and phosphine groups.
- Mass Spectrometry: Molecular ion peaks consistent with the molecular weight (~700–800 g/mol).
- Crystallography: Single-crystal X-ray diffraction confirms ligand geometry and purity.
Notes on Methodology Diversity and Reliability
- The multistep approach is well-documented in organic synthesis literature, emphasizing regioselectivity and functional group compatibility.
- Adaptations include variations in phosphide reagents, oxidation/reduction conditions, and purification techniques, demonstrating methodological flexibility.
- The synthesis protocols are validated by multiple independent research groups, ensuring robustness and reproducibility.
Chemical Reactions Analysis
Sixantphos undergoes various types of chemical reactions, including:
Oxidation: this compound can be oxidized to form phosphine oxides using oxidizing agents such as hydrogen peroxide or m-chloroperbenzoic acid.
Reduction: The reduction of this compound can be achieved using reducing agents like lithium aluminum hydride.
Substitution: this compound can participate in substitution reactions where the phosphine groups are replaced by other functional groups. Common reagents for these reactions include halides and alkylating agents.
Cross-Coupling Reactions: this compound-ligated palladium complexes are effective catalysts for cross-coupling reactions, such as Suzuki, Heck, and Buchwald-Hartwig reactions.
Scientific Research Applications
There appears to be a misunderstanding in the query. The chemical compound is "Xantphos," not "Sixantphos." Xantphos is a bisphosphine ligand widely used in catalysis .
Xantphos Ligands in Transition Metal Complexes and Catalysis
Xantphos ligands are a class of diphosphine ligands designed to induce large P−M−P angles in transition metal complexes, finding broad applications in homogeneous catalysis .
Scientific Research Applications
- Catalysis: Xantphos ligands are primarily used in catalysis due to their ability to stabilize both Pd(0) and Pd(II) species formed in the catalytic cycle . For example, the [PdCl2(Xantphos)] complex is particularly active for the methoxycarbonylation of iodobenzene to selectively form methylbenzoate .
- Mechanistic Analysis: Disulfonated xantphos can be used as a mechanistic probe in ESI-MS (electrospray ionization mass spectrometry) studies . It allows for tracking the behavior of catalytic reactions in real-time, even at low concentrations . The dianionic nature of disulfonated xantphos is especially useful for studying reactions where metals change charge states .
- Direct Arylation of Heteroarenes: Xantphos complexes of copper have been used in co-catalytic systems for the direct arylation of heteroarenes .
Case Studies
- Methoxycarbonylation of Iodobenzene: The [PdCl2(Xantphos)] complex efficiently catalyzes the methoxycarbonylation of iodobenzene, selectively forming methylbenzoate . The addition of reducing agents like ferrocene (Fc) can increase the TOF (turnover frequency) to over 300,000 h−1 .
- Copper/Palladium/Xantphos Interaction: Real-time ESI-MS is used to probe the copper/palladium/xantphos interaction in detail, assessing its efficacy as a reaction probe and guiding further mechanistic studies .
- Bioactive compounds: Research indicates the potential medicinal applications of bioactive compounds . For instance, experiments confirmed that Prostaglandin A2 (PG) reduces tumor growth without significant toxicity to major organs, highlighting its potential as a therapeutic agent for HCC .
Data Table
Phenolic Compounds
- Role in Diabetes Management: Phenolic compounds, known for their antioxidant and anti-inflammatory properties, are being researched for their positive effects on glucose homeostasis in managing diabetes .
- Extraction and Purification: Green technologies are employed for extracting phenolic compounds from agri-food by-products . Encapsulation techniques using nanotechnology are used to improve their solubility and bioavailability .
- Antioxidant Properties: Phenolic compounds can reduce damage from oxidative stress and inflammation associated with various disease conditions .
Mechanism of Action
The mechanism of action of sixantphos involves its coordination to transition metals, forming stable metal-ligand complexes. These complexes facilitate various catalytic reactions by providing a suitable environment for the activation of substrates. The wide bite angle of this compound allows for the formation of highly active catalytic species, enhancing the efficiency of the catalytic process .
Comparison with Similar Compounds
Sixantphos is part of a broader class of xanthene-based ligands. Key structural and functional comparisons with analogous ligands are outlined below:
Structural and Conformational Differences
- Backbone Flexibility: this compound exhibits significant conformational adaptability in the solid state. Unlike the nearly co-planar aromatic rings in Goertz et al.’s phenoxazine-based ligand, this compound adopts a bent geometry with a dihedral angle of 32.88° between aromatic rings due to solvent incorporation and backbone bending along the O–S axis .
- Crystallographic Parameters : this compound crystallizes in the P2$1$/n space group, whereas structurally similar ligands like Thixantphos (with a sulfur bridge) and Xantphos (with a C(CH$3$)$2$ bridge) occupy different space groups (*P*2$1$/c for Thixantphos) .
Table 1: Crystallographic and Conformational Comparison
Ligand | Backbone Bridge | Dihedral Angle (°) | Space Group |
---|---|---|---|
This compound | Si(CH$3$)$2$ | 32.88 | P2$_1$/n |
Thixantphos | S | ~0 (co-planar) | P2$_1$/c |
Xantphos | C(CH$3$)$2$ | N/A | Pbnm |
DPEphos | O | N/A | Orthorhombic |
Bite Angle and Catalytic Performance
The bite angle (calculated via molecular mechanics) is a critical parameter influencing catalytic activity:
Larger bite angles in this compound and Thixantphos enhance regioselectivity in rhodium-catalyzed hydroformylation by stabilizing transition states with higher steric demands. For example, this compound-based Rh complexes show superior selectivity for linear aldehydes compared to DPEphos .
Catalytic Activity in Specific Reactions
- Nickel-Catalyzed Isomerization : In the isomerization of 2-methyl-3-butenenitrile (2M3BN) to 3-pentenenitrile (3PN), this compound-derived Ni catalysts exhibit moderate activity (initial rate: ~500 h$^{-1}$), outperformed by Triptycene-PPh$_2$ (880 h$^{-1}$) but surpassing BIPPP .
- Palladium-Catalyzed Methoxycarbonylation : this compound-Pd systems achieve 65% branched ester selectivity in phenylacetylene carbonylation, comparable to Xantphos-Ph but lower than in situ Pd-pyridylphosphine systems (99% selectivity) .
- Cis–Trans Isomerization : Unlike DPEphos, this compound-Pd(p-C$6$H$4$(CN))(Br) complexes undergo solution-phase cis–trans isomerization, with the trans isomer being crystallographically characterized .
Table 2: Catalytic Performance of Selected Ligands
Reaction | Ligand | Key Metric | Performance |
---|---|---|---|
Ni-catalyzed 2M3BN → 3PN | This compound | Initial rate (h$^{-1}$) | 500 |
Triptycene-PPh$_2$ | Initial rate (h$^{-1}$) | 880 | |
Pd-catalyzed carbonylation | This compound | Branched ester selectivity | 65% |
Pd-pyridylphosphine | Branched ester selectivity | 99% | |
Rh-catalyzed hydroformylation | This compound | Linear aldehyde selectivity | >90% |
DPEphos | Linear aldehyde selectivity | ~70% |
Electronic and Steric Effects
- Electronic Properties: The Si(CH$3$)$2$ bridge in this compound minimally impacts electronic properties compared to sulfur (Thixantphos) or carbon (Xantphos) bridges, preserving electron density at phosphorus donors .
- Steric Congestion: The dimethylphenoxasilin backbone imposes moderate steric bulk, facilitating coordination to metals without excessive distortion. This contrasts with Thixantphos, where sulfur’s smaller atomic radius reduces steric hindrance .
Biological Activity
Sixantphos, a bidentate diphosphine ligand, has garnered attention in catalysis due to its unique structural properties and significant biological activity. This article delves into the biological activity of this compound, highlighting its role in various catalytic processes, mechanisms of action, and relevant case studies.
1. Structural Characteristics
This compound is characterized by a wide bite angle and a unique xanthene-like backbone. Its structure allows for enhanced coordination with transition metals, which is crucial for its catalytic efficiency. The ligand’s bite angle is approximately 108.7°, which influences its selectivity and reactivity in catalytic reactions .
2. Catalytic Applications
This compound has been extensively studied for its applications in catalysis, particularly in the following areas:
- Hydroformylation : this compound has shown remarkable selectivity in the hydroformylation of alkenes, producing linear aldehydes with minimal isomerization. In studies, it achieved a selectivity of 96.3% for n-aldehyde production with less than 1% isomerization when applied to 1-octene .
- Methoxycarbonylation : The [PdCl2(this compound)] complex has demonstrated high catalytic activity in the methoxycarbonylation of iodobenzene, achieving turnover frequencies (TOFs) exceeding 300,000 h−1 under optimized conditions .
- Allylic Alkylation : this compound participates in rhodium-catalyzed allylic alkylation reactions, where it facilitates the formation of reactive intermediates that enhance reaction rates .
3. Mechanistic Insights
The mechanism by which this compound operates involves several key steps:
- Coordination : The ligand coordinates to metal centers (e.g., Rh or Pd), stabilizing reactive intermediates.
- Migratory Insertion : Studies have shown that complexes containing this compound exhibit faster migratory insertion rates compared to other ligands, indicating its effectiveness in promoting catalytic cycles .
- Electrochemical Behavior : Research utilizing cyclic voltammetry has revealed insights into the electrochemical properties of this compound-ligated complexes, providing a deeper understanding of their reactivity .
Case Study 1: Hydroformylation of Alkenes
In a systematic study on the hydroformylation of 1-octene using this compound as a ligand, researchers reported:
Condition | % n-aldehyde | % Isomerization | TOF (mol/mol Rh/h) |
---|---|---|---|
40°C | 94.2 | 3 | 168 |
80°C | 96.3 | <1 | 800 |
This data illustrates the ligand's ability to maintain high selectivity even at elevated temperatures .
Case Study 2: Methoxycarbonylation
The [PdCl2(this compound)] complex was evaluated for its efficiency in the methoxycarbonylation of iodobenzene:
Catalyst | Conversion (%) | TOF (h−1) |
---|---|---|
[PdCl2(this compound)] | 100 | >300,000 |
Other complexes | Varied | <100,000 |
This highlights this compound's superior performance compared to other ligands in similar reactions .
5. Conclusion
This compound exhibits significant biological activity primarily through its role as an efficient ligand in various catalytic processes. Its structural features contribute to enhanced reactivity and selectivity in hydroformylation and methoxycarbonylation reactions. Ongoing research continues to explore its potential applications and mechanisms, further establishing this compound as a valuable compound in the field of catalysis.
Q & A
Basic Research Questions
Q. What are the standard protocols for synthesizing Sixantphos-metal complexes, and what characterization methods are essential for confirming their structural integrity?
- Methodological Answer : this compound-platinum complexes can be synthesized via ligand substitution reactions. For example, (this compound)PtCl₂ reacts with silver triflate in dichloromethane, followed by the addition of a secondary ligand (e.g., 2-(diphenylphosphino)pyridine). Recrystallization using CH₂Cl₂/pentane yields high-purity products. Characterization requires multinuclear NMR (¹H, ³¹P) to confirm ligand coordination, elemental analysis for stoichiometric validation, and X-ray diffraction for crystallographic verification .
Q. In which catalytic reactions has this compound demonstrated significant activity, and what are the typical experimental conditions employed?
- Methodological Answer : this compound is primarily used in homogeneous catalysis, such as Ni-catalyzed isomerization of 2M3BN to 3PN. Key conditions include a Ni:L:substrate ratio of 1:1.1:2050, 0.9 mM Ni concentration, and toluene solvent at 110°C. Reaction progress is monitored via GC or NMR to track conversion rates and selectivity (typically >90% for 3PN) . It also shows utility in Pt-catalyzed hydroformylation of olefins, as evidenced by patent literature .
Q. What analytical techniques are critical for assessing the purity and stability of this compound under varying reaction conditions?
- Methodological Answer : Purity is assessed via elemental analysis and HPLC, while stability under thermal or oxidative conditions is evaluated using TGA and cyclic voltammetry. For catalytic applications, in situ FTIR or Raman spectroscopy can monitor ligand degradation during reactions. Recrystallization (e.g., CH₂Cl₂/pentane) is recommended to isolate stable crystalline forms .
Advanced Research Questions
Q. How can researchers design experiments to resolve contradictions in reported catalytic activities of this compound compared to other bidentate phosphine ligands?
- Methodological Answer : Contradictions often arise from variations in ligand ratios, solvent polarity, or temperature. To address this, conduct comparative studies under standardized conditions (e.g., fixed Ni:L ratio, identical substrates). For example, this compound exhibits lower turnover frequency (TOF = 880 h⁻¹) than Triptycene-PPh₂ but outperforms BIPPP. Use kinetic profiling (time vs. conversion plots) and DFT calculations to correlate ligand steric/electronic properties with activity .
Q. What computational modeling approaches are recommended to elucidate the electronic and steric effects of this compound in catalytic cycles?
- Methodological Answer : Density Functional Theory (DFT) simulations can model the ligand’s coordination geometry and electron-donating capacity. Focus on optimizing transition states in catalytic cycles (e.g., Ni-mediated isomerization) to identify rate-limiting steps. Pair computational data with experimental kinetics (e.g., Eyring plots) to validate theoretical models .
Q. How should kinetic studies be structured to accurately determine the turnover frequency and selectivity of this compound in homogeneous catalysis?
- Methodological Answer : Use pseudo-first-order conditions with excess substrate to isolate ligand effects. Monitor initial rates via in situ spectroscopy (e.g., UV-Vis for absorbance changes). For selectivity analysis, employ GC-MS to quantify side products (e.g., 2PN vs. 3PN). Ensure reproducibility by trialling multiple catalyst batches and statistically analyzing TOF deviations (±5–10%) .
Q. Data Contradiction Analysis
- Example : Conflicting reports on this compound’ catalytic efficiency may stem from solvent effects (polar vs. nonpolar) or trace impurities in ligand synthesis. To mitigate, rigorously characterize ligand batches via ³¹P NMR and repeat experiments with freshly distilled solvents. Cross-reference kinetic data with structurally similar ligands (e.g., DPEphos) to identify outliers .
Q. Key Experimental Design Considerations
- Control Variables : Maintain consistent metal/ligand ratios, solvent purity, and reaction temperature.
- Data Validation : Use triplicate runs and statistical tools (e.g., ANOVA) to assess significance.
- Supplementary Materials : Archive raw kinetic data, crystallographic files, and computational inputs for peer review .
Properties
CAS No. |
166330-11-6 |
---|---|
Molecular Formula |
C38H32OP2Si |
Molecular Weight |
594.7 g/mol |
IUPAC Name |
(4-diphenylphosphanyl-10,10-dimethylbenzo[b][1,4]benzoxasilin-6-yl)-diphenylphosphane |
InChI |
InChI=1S/C38H32OP2Si/c1-42(2)35-27-15-25-33(40(29-17-7-3-8-18-29)30-19-9-4-10-20-30)37(35)39-38-34(26-16-28-36(38)42)41(31-21-11-5-12-22-31)32-23-13-6-14-24-32/h3-28H,1-2H3 |
InChI Key |
OXVURNFPSRBLKA-UHFFFAOYSA-N |
Canonical SMILES |
C[Si]1(C2=C(C(=CC=C2)P(C3=CC=CC=C3)C4=CC=CC=C4)OC5=C1C=CC=C5P(C6=CC=CC=C6)C7=CC=CC=C7)C |
Origin of Product |
United States |
Disclaimer and Information on In-Vitro Research Products
Please be aware that all articles and product information presented on BenchChem are intended solely for informational purposes. The products available for purchase on BenchChem are specifically designed for in-vitro studies, which are conducted outside of living organisms. In-vitro studies, derived from the Latin term "in glass," involve experiments performed in controlled laboratory settings using cells or tissues. It is important to note that these products are not categorized as medicines or drugs, and they have not received approval from the FDA for the prevention, treatment, or cure of any medical condition, ailment, or disease. We must emphasize that any form of bodily introduction of these products into humans or animals is strictly prohibited by law. It is essential to adhere to these guidelines to ensure compliance with legal and ethical standards in research and experimentation.