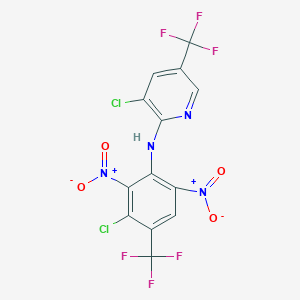
Fluazinam
Overview
Description
Fluazinam is a broad-spectrum fungicide widely used in agriculture. It belongs to the chemical class of diarylamines and is specifically classified as an arylaminopyridine. The chemical name of this compound is 3-chloro-N-(3-chloro-2,6-dinitro-4-trifluoromethylphenyl)-5-trifluoromethyl-2-pyridinamine . It was first described in 1992 and developed by researchers at the Japanese company Ishihara Sangyo Kaisha .
Scientific Research Applications
Fluazinam has a wide range of scientific research applications:
Chemistry: It is used as a model compound to study the reactivity of diarylamines and arylaminopyridines.
Mechanism of Action
Target of Action
Fluazinam is a broad-spectrum fungicide that primarily targets a wide range of fungal pathogens . It is particularly effective against diseases such as grey mold (Botrytis cinerea), white mold (Sclerotinia sclerotiorum), late blight (Phytophthora infestans), and powdery mildew (Erysiphe spp.) . The compound’s primary target is the mitochondria in fungal cells .
Mode of Action
This compound exerts its antifungal effect through a unique mode of action that involves multiple pathways . Specifically, it triggers oxidative stress in fungal cells by inducing reactive oxygen species (ROS) accumulation and caspase activation . This leads to cellular damage and eventual cell death . This compound is a protectant fungicide, but is neither systemic nor curative . It acts by inhibiting the germination of spores and the development of infection structures .
Biochemical Pathways
This compound interrupts the pathogen cell’s energy production process by an uncoupling effect on oxidative phosphorylation . This disruption of the normal functioning of the mitochondria leads to the inhibition of energy production and eventual cell death . Additionally, this compound causes fungal membrane permeabilization and protein carbonylation, which further exacerbate oxidative stress and hasten cell death .
Pharmacokinetics
This compound exhibits excellent in vitro fungicidal activity on both mycelial growth and spore germination . . The compound’s pharmacokinetic properties, such as its distribution and metabolism, are influenced by these interactions with its targets.
Result of Action
The result of this compound’s action is the significant inhibition of fungal growth and spore germination . It causes cellular damage, leading to the death of the fungal cells . Interestingly, this compound especially damages vacuole structures, causing the redistribution of vacuole substances . This leads to the effective control of various fungal diseases in crops .
Action Environment
This compound has a low aqueous solubility and a low volatility . Its toxicity to terrestrial species is low to moderate but is more of a concern for aquatic species . Therefore, the environmental factors such as soil type, water pH, and presence of aquatic species can influence the compound’s action, efficacy, and stability .
Future Directions
The Fluazinam market is expected to grow in the future . It is a highly effective fungicide with broad-spectrum activities and has a high efficacy in inhibiting the growth of many important pathogenic fungi . Evaluating the efficacy of this compound and analyzing the transcriptome data under this compound stress potentially provide a new agent for prevention and control of pests, and also preliminary research results for exploring the mechanism of action of this compound .
Biochemical Analysis
Biochemical Properties
Fluazinam plays a crucial role in biochemical reactions by uncoupling oxidative phosphorylation pathways. This fungicide interacts with various enzymes and proteins involved in cellular respiration. Specifically, this compound inhibits the activity of mitochondrial complex I, leading to the disruption of the electron transport chain and a decrease in adenosine triphosphate production. This interaction results in the accumulation of reactive oxygen species, causing oxidative stress and cell death .
Cellular Effects
This compound affects various types of cells and cellular processes. In plants, this compound promotes the production of metabolites and antioxidants, enhances membrane integrity, and adjusts the redox status of treated plants. These effects lead to improved physiological status and increased yield traits. Additionally, this compound-treated plants exhibit ultrastructural changes, such as an increase in chloroplast starch granules, a giant nucleus, and an elevated number of mitochondria .
Molecular Mechanism
The molecular mechanism of this compound involves its binding interactions with mitochondrial complex I, leading to enzyme inhibition. By disrupting the electron transport chain, this compound causes a decrease in adenosine triphosphate production and an increase in reactive oxygen species. This oxidative stress results in cellular damage and ultimately cell death. This compound’s ability to uncouple oxidative phosphorylation pathways is central to its fungitoxicity .
Temporal Effects in Laboratory Settings
In laboratory settings, the effects of this compound change over time. This compound exhibits long-term effects when applied as a protectant prior to disease onset. Its stability and degradation in various environments, such as crops, water, and soil, have been studied. This compound residues have been detected in these environments, posing potential risks to environmental and human safety. Long-term exposure to this compound can lead to dermatitis and occupational asthma .
Dosage Effects in Animal Models
The effects of this compound vary with different dosages in animal models. Studies have shown that this compound does not cause toxic effects on tested plants at recommended doses. Higher doses can lead to temporary declines in shoot weights and lengths. In animal models, this compound’s toxic or adverse effects at high doses have not been extensively studied, but its classification as a low-toxicity fungicide suggests minimal adverse effects at recommended dosages .
Metabolic Pathways
This compound is involved in metabolic pathways related to oxidative phosphorylation. By inhibiting mitochondrial complex I, this compound disrupts the electron transport chain and decreases adenosine triphosphate production. This disruption affects metabolic flux and metabolite levels, leading to oxidative stress and cellular damage. This compound’s interaction with enzymes and cofactors in these pathways is central to its fungitoxicity .
Transport and Distribution
This compound is transported and distributed within cells and tissues through various mechanisms. It interacts with transporters and binding proteins that facilitate its localization and accumulation. This compound’s ability to penetrate plant tissues and reach target sites is crucial for its effectiveness as a fungicide. Its distribution within cells and tissues influences its activity and function .
Subcellular Localization
This compound’s subcellular localization is primarily within the mitochondria, where it exerts its effects on mitochondrial complex I. The targeting signals and post-translational modifications that direct this compound to specific compartments or organelles are essential for its activity. This compound’s localization within the mitochondria is central to its mechanism of action and fungitoxicity .
Preparation Methods
Chemical Reactions Analysis
Fluazinam undergoes several types of chemical reactions, including:
Oxidation: this compound can be oxidized to form various oxidation products.
Reduction: Reduction reactions can lead to the formation of amine derivatives.
Substitution: this compound can undergo substitution reactions where functional groups are replaced by other groups.
Common reagents used in these reactions include oxidizing agents like hydrogen peroxide, reducing agents like sodium borohydride, and various nucleophiles for substitution reactions . The major products formed from these reactions depend on the specific conditions and reagents used .
Comparison with Similar Compounds
Fluazinam is unique among fungicides due to its broad-spectrum activity and low toxicity to mammals. Similar compounds include:
Chlorothalonil: Another broad-spectrum fungicide with a different mode of action.
Mancozeb: A fungicide that works by inhibiting enzyme activity in fungal cells.
Azoxystrobin: A fungicide that inhibits mitochondrial respiration in fungi.
This compound stands out due to its unique mode of action and its effectiveness against a wide range of fungal pathogens .
Properties
IUPAC Name |
3-chloro-N-[3-chloro-2,6-dinitro-4-(trifluoromethyl)phenyl]-5-(trifluoromethyl)pyridin-2-amine | |
---|---|---|
Source | PubChem | |
URL | https://pubchem.ncbi.nlm.nih.gov | |
Description | Data deposited in or computed by PubChem | |
InChI |
InChI=1S/C13H4Cl2F6N4O4/c14-6-1-4(12(16,17)18)3-22-11(6)23-9-7(24(26)27)2-5(13(19,20)21)8(15)10(9)25(28)29/h1-3H,(H,22,23) | |
Source | PubChem | |
URL | https://pubchem.ncbi.nlm.nih.gov | |
Description | Data deposited in or computed by PubChem | |
InChI Key |
UZCGKGPEKUCDTF-UHFFFAOYSA-N | |
Source | PubChem | |
URL | https://pubchem.ncbi.nlm.nih.gov | |
Description | Data deposited in or computed by PubChem | |
Canonical SMILES |
C1=C(C=NC(=C1Cl)NC2=C(C=C(C(=C2[N+](=O)[O-])Cl)C(F)(F)F)[N+](=O)[O-])C(F)(F)F | |
Source | PubChem | |
URL | https://pubchem.ncbi.nlm.nih.gov | |
Description | Data deposited in or computed by PubChem | |
Molecular Formula |
C13H4Cl2F6N4O4 | |
Source | PubChem | |
URL | https://pubchem.ncbi.nlm.nih.gov | |
Description | Data deposited in or computed by PubChem | |
DSSTOX Substance ID |
DTXSID7032551 | |
Record name | Fluazinam | |
Source | EPA DSSTox | |
URL | https://comptox.epa.gov/dashboard/DTXSID7032551 | |
Description | DSSTox provides a high quality public chemistry resource for supporting improved predictive toxicology. | |
Molecular Weight |
465.09 g/mol | |
Source | PubChem | |
URL | https://pubchem.ncbi.nlm.nih.gov | |
Description | Data deposited in or computed by PubChem | |
Physical Description |
Light yellow solid; [HSDB] Omega 500F: Yellow liquid with a pungent odor (40% active ingredient); [Reference #2] | |
Record name | Fluazinam | |
Source | Haz-Map, Information on Hazardous Chemicals and Occupational Diseases | |
URL | https://haz-map.com/Agents/1226 | |
Description | Haz-Map® is an occupational health database designed for health and safety professionals and for consumers seeking information about the adverse effects of workplace exposures to chemical and biological agents. | |
Explanation | Copyright (c) 2022 Haz-Map(R). All rights reserved. Unless otherwise indicated, all materials from Haz-Map are copyrighted by Haz-Map(R). No part of these materials, either text or image may be used for any purpose other than for personal use. Therefore, reproduction, modification, storage in a retrieval system or retransmission, in any form or by any means, electronic, mechanical or otherwise, for reasons other than personal use, is strictly prohibited without prior written permission. | |
Solubility |
Solubility in n-hexane 12, acetone 470, toluene 410, diethyl ether 320, dichloromethane 330, ethanol 150 (all in g/L, 20 °C), Solubility in methanol 162 g/L, dichloroethane 485 g/L, ethyl acetate 624 g/L, In water, 1.35X10-1 mg/L at 20 °C | |
Record name | FLUAZINAM | |
Source | Hazardous Substances Data Bank (HSDB) | |
URL | https://pubchem.ncbi.nlm.nih.gov/source/hsdb/7264 | |
Description | The Hazardous Substances Data Bank (HSDB) is a toxicology database that focuses on the toxicology of potentially hazardous chemicals. It provides information on human exposure, industrial hygiene, emergency handling procedures, environmental fate, regulatory requirements, nanomaterials, and related areas. The information in HSDB has been assessed by a Scientific Review Panel. | |
Density |
1.259 g/mL at 25 °C | |
Record name | FLUAZINAM | |
Source | Hazardous Substances Data Bank (HSDB) | |
URL | https://pubchem.ncbi.nlm.nih.gov/source/hsdb/7264 | |
Description | The Hazardous Substances Data Bank (HSDB) is a toxicology database that focuses on the toxicology of potentially hazardous chemicals. It provides information on human exposure, industrial hygiene, emergency handling procedures, environmental fate, regulatory requirements, nanomaterials, and related areas. The information in HSDB has been assessed by a Scientific Review Panel. | |
Vapor Pressure |
0.000056 [mmHg], 5.6X10-5 mm Hg at 25 °C | |
Record name | Fluazinam | |
Source | Haz-Map, Information on Hazardous Chemicals and Occupational Diseases | |
URL | https://haz-map.com/Agents/1226 | |
Description | Haz-Map® is an occupational health database designed for health and safety professionals and for consumers seeking information about the adverse effects of workplace exposures to chemical and biological agents. | |
Explanation | Copyright (c) 2022 Haz-Map(R). All rights reserved. Unless otherwise indicated, all materials from Haz-Map are copyrighted by Haz-Map(R). No part of these materials, either text or image may be used for any purpose other than for personal use. Therefore, reproduction, modification, storage in a retrieval system or retransmission, in any form or by any means, electronic, mechanical or otherwise, for reasons other than personal use, is strictly prohibited without prior written permission. | |
Record name | FLUAZINAM | |
Source | Hazardous Substances Data Bank (HSDB) | |
URL | https://pubchem.ncbi.nlm.nih.gov/source/hsdb/7264 | |
Description | The Hazardous Substances Data Bank (HSDB) is a toxicology database that focuses on the toxicology of potentially hazardous chemicals. It provides information on human exposure, industrial hygiene, emergency handling procedures, environmental fate, regulatory requirements, nanomaterials, and related areas. The information in HSDB has been assessed by a Scientific Review Panel. | |
Mechanism of Action |
Fluazinam is a lipophilic weak acid with strong uncoupling activity on mitochondria in vitro. | |
Record name | FLUAZINAM | |
Source | Hazardous Substances Data Bank (HSDB) | |
URL | https://pubchem.ncbi.nlm.nih.gov/source/hsdb/7264 | |
Description | The Hazardous Substances Data Bank (HSDB) is a toxicology database that focuses on the toxicology of potentially hazardous chemicals. It provides information on human exposure, industrial hygiene, emergency handling procedures, environmental fate, regulatory requirements, nanomaterials, and related areas. The information in HSDB has been assessed by a Scientific Review Panel. | |
Color/Form |
Light yellow crystals | |
CAS No. |
79622-59-6 | |
Record name | Fluazinam | |
Source | CAS Common Chemistry | |
URL | https://commonchemistry.cas.org/detail?cas_rn=79622-59-6 | |
Description | CAS Common Chemistry is an open community resource for accessing chemical information. Nearly 500,000 chemical substances from CAS REGISTRY cover areas of community interest, including common and frequently regulated chemicals, and those relevant to high school and undergraduate chemistry classes. This chemical information, curated by our expert scientists, is provided in alignment with our mission as a division of the American Chemical Society. | |
Explanation | The data from CAS Common Chemistry is provided under a CC-BY-NC 4.0 license, unless otherwise stated. | |
Record name | Fluazinam [ISO] | |
Source | ChemIDplus | |
URL | https://pubchem.ncbi.nlm.nih.gov/substance/?source=chemidplus&sourceid=0079622596 | |
Description | ChemIDplus is a free, web search system that provides access to the structure and nomenclature authority files used for the identification of chemical substances cited in National Library of Medicine (NLM) databases, including the TOXNET system. | |
Record name | Fluazinam | |
Source | EPA DSSTox | |
URL | https://comptox.epa.gov/dashboard/DTXSID7032551 | |
Description | DSSTox provides a high quality public chemistry resource for supporting improved predictive toxicology. | |
Record name | 2-Pyridinamine, 3-chloro-N-[3-chloro-2,6-dinitro-4-(trifluoromethyl)phenyl]-5-(trifluoromethyl) | |
Source | European Chemicals Agency (ECHA) | |
URL | https://echa.europa.eu/substance-information/-/substanceinfo/100.114.073 | |
Description | The European Chemicals Agency (ECHA) is an agency of the European Union which is the driving force among regulatory authorities in implementing the EU's groundbreaking chemicals legislation for the benefit of human health and the environment as well as for innovation and competitiveness. | |
Explanation | Use of the information, documents and data from the ECHA website is subject to the terms and conditions of this Legal Notice, and subject to other binding limitations provided for under applicable law, the information, documents and data made available on the ECHA website may be reproduced, distributed and/or used, totally or in part, for non-commercial purposes provided that ECHA is acknowledged as the source: "Source: European Chemicals Agency, http://echa.europa.eu/". Such acknowledgement must be included in each copy of the material. ECHA permits and encourages organisations and individuals to create links to the ECHA website under the following cumulative conditions: Links can only be made to webpages that provide a link to the Legal Notice page. | |
Record name | FLUAZINAM | |
Source | FDA Global Substance Registration System (GSRS) | |
URL | https://gsrs.ncats.nih.gov/ginas/app/beta/substances/0P91PCK33Q | |
Description | The FDA Global Substance Registration System (GSRS) enables the efficient and accurate exchange of information on what substances are in regulated products. Instead of relying on names, which vary across regulatory domains, countries, and regions, the GSRS knowledge base makes it possible for substances to be defined by standardized, scientific descriptions. | |
Explanation | Unless otherwise noted, the contents of the FDA website (www.fda.gov), both text and graphics, are not copyrighted. They are in the public domain and may be republished, reprinted and otherwise used freely by anyone without the need to obtain permission from FDA. Credit to the U.S. Food and Drug Administration as the source is appreciated but not required. | |
Record name | FLUAZINAM | |
Source | Hazardous Substances Data Bank (HSDB) | |
URL | https://pubchem.ncbi.nlm.nih.gov/source/hsdb/7264 | |
Description | The Hazardous Substances Data Bank (HSDB) is a toxicology database that focuses on the toxicology of potentially hazardous chemicals. It provides information on human exposure, industrial hygiene, emergency handling procedures, environmental fate, regulatory requirements, nanomaterials, and related areas. The information in HSDB has been assessed by a Scientific Review Panel. | |
Melting Point |
115-117 °C | |
Record name | FLUAZINAM | |
Source | Hazardous Substances Data Bank (HSDB) | |
URL | https://pubchem.ncbi.nlm.nih.gov/source/hsdb/7264 | |
Description | The Hazardous Substances Data Bank (HSDB) is a toxicology database that focuses on the toxicology of potentially hazardous chemicals. It provides information on human exposure, industrial hygiene, emergency handling procedures, environmental fate, regulatory requirements, nanomaterials, and related areas. The information in HSDB has been assessed by a Scientific Review Panel. | |
Retrosynthesis Analysis
AI-Powered Synthesis Planning: Our tool employs the Template_relevance Pistachio, Template_relevance Bkms_metabolic, Template_relevance Pistachio_ringbreaker, Template_relevance Reaxys, Template_relevance Reaxys_biocatalysis model, leveraging a vast database of chemical reactions to predict feasible synthetic routes.
One-Step Synthesis Focus: Specifically designed for one-step synthesis, it provides concise and direct routes for your target compounds, streamlining the synthesis process.
Accurate Predictions: Utilizing the extensive PISTACHIO, BKMS_METABOLIC, PISTACHIO_RINGBREAKER, REAXYS, REAXYS_BIOCATALYSIS database, our tool offers high-accuracy predictions, reflecting the latest in chemical research and data.
Strategy Settings
Precursor scoring | Relevance Heuristic |
---|---|
Min. plausibility | 0.01 |
Model | Template_relevance |
Template Set | Pistachio/Bkms_metabolic/Pistachio_ringbreaker/Reaxys/Reaxys_biocatalysis |
Top-N result to add to graph | 6 |
Feasible Synthetic Routes
Disclaimer and Information on In-Vitro Research Products
Please be aware that all articles and product information presented on BenchChem are intended solely for informational purposes. The products available for purchase on BenchChem are specifically designed for in-vitro studies, which are conducted outside of living organisms. In-vitro studies, derived from the Latin term "in glass," involve experiments performed in controlled laboratory settings using cells or tissues. It is important to note that these products are not categorized as medicines or drugs, and they have not received approval from the FDA for the prevention, treatment, or cure of any medical condition, ailment, or disease. We must emphasize that any form of bodily introduction of these products into humans or animals is strictly prohibited by law. It is essential to adhere to these guidelines to ensure compliance with legal and ethical standards in research and experimentation.