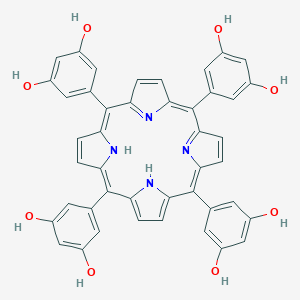
5,10,15,20-Tetrakis(3,5-dihydroxyphenyl)-21H,23H-porphine
- Click on QUICK INQUIRY to receive a quote from our team of experts.
- With the quality product at a COMPETITIVE price, you can focus more on your research.
Overview
Description
The compound of interest, 5,10,15,20-Tetrakis(3,5-dihydroxyphenyl)-21H,23H-porphine, is a derivative of porphyrin, a class of organic compounds known for their rich photophysical properties and potential applications in various fields, including photodynamic therapy and as sensors. While the specific compound is not directly mentioned in the provided papers, the derivatives of porphyrins discussed in these papers offer insights into the structural and functional versatility of porphyrins, which can be extrapolated to understand the compound .
Synthesis Analysis
The synthesis of porphyrin derivatives often involves the direct reaction from corresponding aldehydes. For instance, brominated and iodinated derivatives of 5,10,15,20-tetrakis(3-hydroxyphenyl)porphyrin were synthesized directly from the corresponding aldehydes . Another derivative, 5,10,15,20-Tetrakis(2-amino-6-methoxycarbonylphenyl)porphyrin, was synthesized, and its atropisomers were separated, indicating the complexity and specificity of synthesis methods for these compounds . These methods highlight the synthetic versatility and the ability to introduce various functional groups onto the porphyrin core.
Molecular Structure Analysis
The molecular structure of porphyrin derivatives is characterized by the presence of a porphyrin core with various substituents attached to the phenyl rings. The rotation of the bond between the phenyl and porphyrin rings can be restricted, as seen in the case of 5,10,15,20-Tetrakis(2-amino-6-methoxycarbonylphenyl)porphyrin, where thermal isomerization was prohibited . The structure of these compounds can be confirmed using techniques such as NMR spectroscopy and mass spectrometry .
Chemical Reactions Analysis
Porphyrin derivatives undergo various chemical reactions, including photobleaching, which is a light-induced decomposition process. For example, 5,10,15,20-Tetrakis(m-hydroxyphenyl)porphyrin (m-THPP) yielded quinonoid porphyrins upon irradiation in aqueous methanol . These reactions are significant in understanding the stability and reactivity of porphyrin derivatives under different conditions.
Physical and Chemical Properties Analysis
The physical and chemical properties of porphyrin derivatives are influenced by their substituents. For instance, the photophysical and photochemical properties, such as singlet oxygen formation quantum yields and photobleaching, were measured for brominated and iodinated derivatives . The basicity constants and the existence ranges of protonated forms of the compounds were determined using spectrophotometric titration . Additionally, thermal and electrical properties, such as thermal stability and electrical conductivity, were studied for a cobalt(III) complex of a porphyrin derivative .
Case Studies
Several case studies demonstrate the potential applications of porphyrin derivatives. The cytotoxicity assays on WiDr and A375 tumor cell lines showed that certain derivatives have significant cytotoxic effects, which could be leveraged in cancer treatment . The binding properties of fluorinated porphyrins suggest their use as optical sensors due to their affinity for fluorinated substrates . Moreover, a water-soluble porphyrin derivative was used as a highly sensitive chromogenic reagent for detecting alkaline phosphatase enzyme activity .
Scientific Research Applications
Interaction with Single-Walled Carbon Nanotubes
5,10,15,20-Tetrakis(hexadecyloxyphenyl)-21H,23H-porphine demonstrates selective interactions with semiconducting single-walled carbon nanotubes (SWNTs). These interactions are predominantly noncovalent and result in an enrichment of semiconducting SWNTs in solubilized samples, offering potential applications in nanotechnology and materials science (Li et al., 2004).
Reaction with Nitrogen Dioxide
The response of similar porphyrins, such as 5,10,15,20-tetrakis(3,4-bis[ethylhexyloxy]phenyl)-21H,23H-porphine, to nitrogen dioxide (NO2) has been studied. This includes changes in absorption spectrum upon exposure to NO2, which could be relevant for environmental monitoring and sensor technology (Mcnaughton et al., 2006).
Surface Ion Association
Studies on the surface ion association of porphyrins like 5,10,15,20-tetrakis(1-methyl-4-pyridyl)-21H,23H-porphine have demonstrated their ability to form ion pairs with hexacyanoferrate ions. This has implications for electrochemical applications and the study of ion association dynamics (Zhang, Lever, & Pietro, 1997).
Fluorescence Probe for Phospholipids
5,10,15,20-Tetrakis(3-hydroxyphenyl)-21H,23H-porphine has been investigated as a potential fluorescence probe for detecting phospholipids. Its ability to enhance emission in the presence of lipids suggests applications in biochemical research and diagnostics (Ibrahim et al., 2011).
Chirality Amplification in Porphyrin Assemblies
Research has shown that achiral porphyrin derivatives, including ones similar to 5,10,15,20-tetrakis(4-hydroxyphenyl)-21H,23H-porphine, can exhibit macroscopic supramolecular chirality when spread onto an air/water interface. This unexpected chirality amplification has potential applications in materials science and molecular engineering (Chen et al., 2006).
Interfacial Ion-Association Adsorption
Studies on 5,10,15,20-tetrakis(4-sulfonatophenyl)-21H,23H-porphine have revealed its interfacial ion-association adsorption properties. These findings are significant for understanding the interactions of porphyrins at liquid-liquid interfaces, with potential implications in electrochemistry and surface science (Saitoh & Watarai, 1997).
Safety and Hazards
The compound is labeled with the GHS07 pictogram, indicating that it may cause skin irritation, eye irritation, and may cause respiratory irritation . Precautionary measures include avoiding breathing dust/fume/gas/mist/vapors/spray, wearing protective gloves/protective clothing/eye protection/face protection, and if swallowed, calling a poison center or doctor if feeling unwell .
properties
IUPAC Name |
5-[10,15,20-tris(3,5-dihydroxyphenyl)-21,23-dihydroporphyrin-5-yl]benzene-1,3-diol |
Source
|
---|---|---|
Source | PubChem | |
URL | https://pubchem.ncbi.nlm.nih.gov | |
Description | Data deposited in or computed by PubChem | |
InChI |
InChI=1S/C44H30N4O8/c49-25-9-21(10-26(50)17-25)41-33-1-2-34(45-33)42(22-11-27(51)18-28(52)12-22)36-5-6-38(47-36)44(24-15-31(55)20-32(56)16-24)40-8-7-39(48-40)43(37-4-3-35(41)46-37)23-13-29(53)19-30(54)14-23/h1-20,45,48-56H |
Source
|
Source | PubChem | |
URL | https://pubchem.ncbi.nlm.nih.gov | |
Description | Data deposited in or computed by PubChem | |
InChI Key |
KNNIRTCDHGJGJA-UHFFFAOYSA-N |
Source
|
Source | PubChem | |
URL | https://pubchem.ncbi.nlm.nih.gov | |
Description | Data deposited in or computed by PubChem | |
Canonical SMILES |
C1=CC2=C(C3=NC(=C(C4=CC=C(N4)C(=C5C=CC(=N5)C(=C1N2)C6=CC(=CC(=C6)O)O)C7=CC(=CC(=C7)O)O)C8=CC(=CC(=C8)O)O)C=C3)C9=CC(=CC(=C9)O)O |
Source
|
Source | PubChem | |
URL | https://pubchem.ncbi.nlm.nih.gov | |
Description | Data deposited in or computed by PubChem | |
Molecular Formula |
C44H30N4O8 |
Source
|
Source | PubChem | |
URL | https://pubchem.ncbi.nlm.nih.gov | |
Description | Data deposited in or computed by PubChem | |
Molecular Weight |
742.7 g/mol |
Source
|
Source | PubChem | |
URL | https://pubchem.ncbi.nlm.nih.gov | |
Description | Data deposited in or computed by PubChem | |
Product Name |
5,10,15,20-Tetrakis(3,5-dihydroxyphenyl)-21H,23H-porphine |
Q & A
Q1: What makes TOH(d)PP a promising candidate for selectively targeting human telomeric repeat-containing RNA (TERRA) G-quadruplexes?
A1: TOH(d)PP demonstrates exceptional selectivity for TERRA G-quadruplexes over DNA G-quadruplexes. This selectivity arises from its unique interaction with the structural features specific to TERRA G4s. Specifically:
- Hyperporphyrin effect activation: TOH(d)PP exhibits a distinct spectral shift and enhanced fluorescence emission only upon binding to TERRA G4s. [] This phenomenon, known as the hyperporphyrin effect, is triggered by the specific structural arrangement of TERRA G4s.
- Interaction with 5' tetrads: Research suggests that TOH(d)PP interacts with the 5' tetrads of two TERRA G4s through a 1:2 sandwich association. [] This interaction is facilitated by the presence of eight hydroxyl groups on the TOH(d)PP molecule.
- Recognition of ribose 2'-OH induced structure: The ribose 2'-OH group in TERRA favors a specific conformation where the loop adenine residue extends towards the G-tetrad plane. [] TOH(d)PP likely interacts with this unique structural motif, further enhancing its selectivity for TERRA over DNA G4s.
Q2: How does the structure of TOH(d)PP contribute to its sensing capabilities?
A2: The molecular structure of TOH(d)PP plays a crucial role in its ability to function as a sensitive material in chemical sensors:
- Porosity and Hydrogen Bonding: The peripheral hydroxyl groups in TOH(d)PP enable the formation of porous thin films. [] These films can effectively trap gaseous analytes, particularly those capable of hydrogen bonding.
- Supramolecular Aggregation: TOH(d)PP molecules can self-assemble into supramolecular aggregates, creating a network of pores within the sensing layer. [] This unique arrangement provides a sensing mechanism not observed in individual molecules, allowing for the detection of gases like carbon monoxide (CO) through entrapment within the porous structure.
Q3: Are there any studies on the aggregation behavior of TOH(d)PP and its closely related compound, 5,10,15,20-tetrakis(m-hydroxyphenyl)chlorin (m-THPC)?
A3: Yes, research indicates that both compounds exhibit distinct aggregation behaviors depending on the solvent environment:
Disclaimer and Information on In-Vitro Research Products
Please be aware that all articles and product information presented on BenchChem are intended solely for informational purposes. The products available for purchase on BenchChem are specifically designed for in-vitro studies, which are conducted outside of living organisms. In-vitro studies, derived from the Latin term "in glass," involve experiments performed in controlled laboratory settings using cells or tissues. It is important to note that these products are not categorized as medicines or drugs, and they have not received approval from the FDA for the prevention, treatment, or cure of any medical condition, ailment, or disease. We must emphasize that any form of bodily introduction of these products into humans or animals is strictly prohibited by law. It is essential to adhere to these guidelines to ensure compliance with legal and ethical standards in research and experimentation.