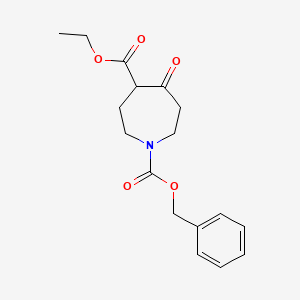
1-Benzyl 4-ethyl 5-oxoazepane-1,4-dicarboxylate
Overview
Description
1-Benzyl 4-ethyl 5-oxoazepane-1,4-dicarboxylate is a versatile small molecule scaffold with the molecular formula C17H21NO5 and a molecular weight of 319.36 g/mol . This compound is characterized by its azepane ring, which is a seven-membered heterocyclic ring containing one nitrogen atom. The presence of both benzyl and ethyl groups, along with two ester functionalities, makes this compound an interesting subject for various chemical studies and applications.
Preparation Methods
The synthesis of 1-Benzyl 4-ethyl 5-oxoazepane-1,4-dicarboxylate typically involves the following steps :
Starting Materials: The synthesis begins with the appropriate azepane derivative and benzyl bromide.
Reaction Conditions: The reaction is carried out under inert atmosphere conditions, typically at temperatures ranging from 2°C to 8°C.
Chemical Reactions Analysis
1-Benzyl 4-ethyl 5-oxoazepane-1,4-dicarboxylate undergoes various chemical reactions, including:
Oxidation: The compound can be oxidized using common oxidizing agents such as potassium permanganate or chromium trioxide.
Reduction: Reduction reactions can be performed using reagents like lithium aluminum hydride or sodium borohydride.
Substitution: Nucleophilic substitution reactions can occur at the benzyl or ethyl positions using reagents like sodium methoxide or potassium tert-butoxide.
The major products formed from these reactions depend on the specific conditions and reagents used. For example, oxidation may yield carboxylic acids, while reduction may produce alcohols.
Scientific Research Applications
1-Benzyl 4-ethyl 5-oxoazepane-1,4-dicarboxylate has several scientific research applications :
Chemistry: It serves as a building block for the synthesis of more complex molecules.
Biology: The compound is used in the study of enzyme interactions and as a potential inhibitor in biochemical assays.
Medicine: Research is ongoing to explore its potential as a pharmaceutical intermediate.
Industry: It is used in the development of new materials and as a reference standard in analytical chemistry.
Mechanism of Action
The mechanism of action of 1-Benzyl 4-ethyl 5-oxoazepane-1,4-dicarboxylate involves its interaction with specific molecular targets and pathways . The compound can act as an inhibitor by binding to active sites of enzymes, thereby blocking their activity. The exact molecular targets and pathways depend on the specific application and context of use.
Comparison with Similar Compounds
1-Benzyl 4-ethyl 5-oxoazepane-1,4-dicarboxylate can be compared with other similar compounds such as :
1-tert-Butyl 4-ethyl 5-oxoazepane-1,4-dicarboxylate: Similar structure but with a tert-butyl group instead of a benzyl group.
1-Benzyl 4-methyl 5-oxoazepane-1,4-dicarboxylate: Similar structure but with a methyl group instead of an ethyl group.
The uniqueness of this compound lies in its specific combination of functional groups, which imparts distinct chemical and biological properties.
Properties
IUPAC Name |
1-O-benzyl 4-O-ethyl 5-oxoazepane-1,4-dicarboxylate | |
---|---|---|
Source | PubChem | |
URL | https://pubchem.ncbi.nlm.nih.gov | |
Description | Data deposited in or computed by PubChem | |
InChI |
InChI=1S/C17H21NO5/c1-2-22-16(20)14-8-10-18(11-9-15(14)19)17(21)23-12-13-6-4-3-5-7-13/h3-7,14H,2,8-12H2,1H3 | |
Source | PubChem | |
URL | https://pubchem.ncbi.nlm.nih.gov | |
Description | Data deposited in or computed by PubChem | |
InChI Key |
YMPFJXQFTCDJOB-UHFFFAOYSA-N | |
Source | PubChem | |
URL | https://pubchem.ncbi.nlm.nih.gov | |
Description | Data deposited in or computed by PubChem | |
Canonical SMILES |
CCOC(=O)C1CCN(CCC1=O)C(=O)OCC2=CC=CC=C2 | |
Source | PubChem | |
URL | https://pubchem.ncbi.nlm.nih.gov | |
Description | Data deposited in or computed by PubChem | |
Molecular Formula |
C17H21NO5 | |
Source | PubChem | |
URL | https://pubchem.ncbi.nlm.nih.gov | |
Description | Data deposited in or computed by PubChem | |
DSSTOX Substance ID |
DTXSID60562093 | |
Record name | 1-Benzyl 4-ethyl 5-oxoazepane-1,4-dicarboxylate | |
Source | EPA DSSTox | |
URL | https://comptox.epa.gov/dashboard/DTXSID60562093 | |
Description | DSSTox provides a high quality public chemistry resource for supporting improved predictive toxicology. | |
Molecular Weight |
319.4 g/mol | |
Source | PubChem | |
URL | https://pubchem.ncbi.nlm.nih.gov | |
Description | Data deposited in or computed by PubChem | |
CAS No. |
31696-09-0 | |
Record name | 1-Benzyl 4-ethyl 5-oxoazepane-1,4-dicarboxylate | |
Source | EPA DSSTox | |
URL | https://comptox.epa.gov/dashboard/DTXSID60562093 | |
Description | DSSTox provides a high quality public chemistry resource for supporting improved predictive toxicology. | |
Synthesis routes and methods I
Procedure details
Synthesis routes and methods II
Procedure details
Synthesis routes and methods III
Procedure details
Disclaimer and Information on In-Vitro Research Products
Please be aware that all articles and product information presented on BenchChem are intended solely for informational purposes. The products available for purchase on BenchChem are specifically designed for in-vitro studies, which are conducted outside of living organisms. In-vitro studies, derived from the Latin term "in glass," involve experiments performed in controlled laboratory settings using cells or tissues. It is important to note that these products are not categorized as medicines or drugs, and they have not received approval from the FDA for the prevention, treatment, or cure of any medical condition, ailment, or disease. We must emphasize that any form of bodily introduction of these products into humans or animals is strictly prohibited by law. It is essential to adhere to these guidelines to ensure compliance with legal and ethical standards in research and experimentation.