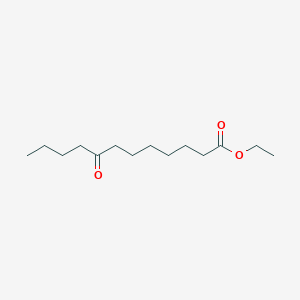
Ethyl 8-oxododecanoate
- Click on QUICK INQUIRY to receive a quote from our team of experts.
- With the quality product at a COMPETITIVE price, you can focus more on your research.
Overview
Description
Ethyl 8-oxododecanoate is an organic compound with the molecular formula C14H26O3. It is a light-colored oil and is known for its applications in various scientific fields. The compound is characterized by the presence of an ester functional group and a ketone group, which contribute to its reactivity and versatility in chemical synthesis .
Preparation Methods
Synthetic Routes and Reaction Conditions: Ethyl 8-oxododecanoate can be synthesized through several methods. One common synthetic route involves the reaction of ethyl 7-chloro-7-formylheptanoate with manganese and butyliodo . Another method involves the nickel-catalyzed synthesis of ketones from alkyl halides and acid chlorides . The reaction typically requires a four-necked reactor, nickel (II) chloride ethylene glycol dimethyl ether complex, 4,4’-di-tert-butyl-2,2’-dipyridyl, manganese powder, and anhydrous N,N-dimethylacetamide. The reaction mixture is stirred under nitrogen flow and cooled to specific temperatures to achieve the desired product .
Industrial Production Methods: Industrial production methods for this compound are similar to laboratory synthesis but are scaled up to accommodate larger quantities. The use of continuous flow reactors and optimized reaction conditions ensures efficient production while maintaining high purity and yield.
Chemical Reactions Analysis
Types of Reactions: Ethyl 8-oxododecanoate undergoes various chemical reactions, including oxidation, reduction, and substitution. The presence of both ester and ketone functional groups allows it to participate in a wide range of reactions.
Common Reagents and Conditions:
Oxidation: Common oxidizing agents such as potassium permanganate or chromium trioxide can be used to oxidize this compound, leading to the formation of carboxylic acids or other oxidized products.
Reduction: Reducing agents like lithium aluminum hydride or sodium borohydride can reduce the ketone group to an alcohol.
Substitution: Nucleophilic substitution reactions can occur at the ester group, leading to the formation of various derivatives.
Major Products Formed: The major products formed from these reactions depend on the specific reagents and conditions used. For example, oxidation can yield carboxylic acids, while reduction can produce alcohols .
Scientific Research Applications
Ethyl 8-oxododecanoate has a wide range of applications in scientific research:
Chemistry: It is used as an intermediate in organic synthesis, particularly in the preparation of more complex molecules.
Biology: The compound is studied for its potential biological activities and interactions with various biomolecules.
Medicine: Research is ongoing to explore its potential therapeutic applications, including its use as a precursor for drug development.
Industry: this compound is used in the production of fragrances, flavors, and other industrial chemicals
Mechanism of Action
The mechanism of action of ethyl 8-oxododecanoate involves its interaction with specific molecular targets and pathways. The ester and ketone groups allow it to participate in various biochemical reactions, potentially affecting enzyme activity and cellular processes. Detailed studies on its mechanism of action are still ongoing, and further research is needed to fully understand its effects .
Comparison with Similar Compounds
- Ethyl 4-oxododecanoate
- Ethyl 6-oxododecanoate
- Ethyl 10-oxododecanoate
Comparison: Ethyl 8-oxododecanoate is unique due to the position of the ketone group on the eighth carbon of the dodecanoate chain. This specific positioning influences its reactivity and the types of reactions it can undergo. Compared to similar compounds, this compound may exhibit different chemical and biological properties, making it valuable for specific applications .
Biological Activity
Ethyl 8-oxododecanoate, also known as ethyl 8-oxo-dodecanoate, is a compound that has garnered interest in various fields of research due to its potential biological activities. This article provides a comprehensive overview of the biological activity of this compound, including its chemical properties, synthesis, and relevant studies that highlight its effects in biological systems.
This compound has the molecular formula C14H26O3 and a molecular weight of approximately 242.35 g/mol. It is characterized by the presence of an oxo group at the 8-position of the dodecanoate chain, which contributes to its reactivity and biological activity. The compound is typically synthesized through esterification reactions involving dodecanoic acid derivatives.
Property | Value |
---|---|
Molecular Formula | C₁₄H₂₆O₃ |
Molecular Weight | 242.35 g/mol |
CAS Number | 97037-83-7 |
Physical State | Liquid |
Synthesis
The synthesis of this compound can be achieved through various organic reactions. One common method involves the reaction of dodecanoic acid with ethyl alcohol in the presence of an acid catalyst. This process can be optimized to yield high purity and yield of the desired ester.
Antimicrobial Properties
Research has indicated that this compound exhibits antimicrobial activity against various pathogens. A study demonstrated its effectiveness against Candida albicans , a common yeast pathogen, where it was noted to inhibit growth at specific concentrations. The minimum inhibitory concentration (MIC) was determined using standard broth microdilution methods, showing significant antifungal properties.
Cytotoxic Effects
In addition to its antimicrobial properties, this compound has been evaluated for cytotoxic effects on cancer cell lines. In vitro studies have shown that this compound can induce apoptosis in certain cancer cell types, suggesting potential applications in cancer therapy. The mechanism appears to involve oxidative stress pathways and mitochondrial dysfunction.
Case Studies
- Antifungal Activity : A study reported that this compound had an MIC against C. albicans at concentrations ranging from 50 to 100 μg/mL, indicating its potential as an antifungal agent in therapeutic applications .
- Cytotoxicity in Cancer Cells : In a controlled experiment involving human cancer cell lines, treatment with this compound resulted in a dose-dependent decrease in cell viability, with significant effects observed at concentrations above 100 μM .
The biological activity of this compound is thought to stem from its ability to interact with cellular membranes and influence metabolic pathways. Its oxo group may facilitate redox reactions leading to increased reactive oxygen species (ROS), which can trigger apoptosis in susceptible cells.
Properties
IUPAC Name |
ethyl 8-oxododecanoate |
Source
|
---|---|---|
Source | PubChem | |
URL | https://pubchem.ncbi.nlm.nih.gov | |
Description | Data deposited in or computed by PubChem | |
InChI |
InChI=1S/C14H26O3/c1-3-5-10-13(15)11-8-6-7-9-12-14(16)17-4-2/h3-12H2,1-2H3 |
Source
|
Source | PubChem | |
URL | https://pubchem.ncbi.nlm.nih.gov | |
Description | Data deposited in or computed by PubChem | |
InChI Key |
MTPJRQYMPYHMKN-UHFFFAOYSA-N |
Source
|
Source | PubChem | |
URL | https://pubchem.ncbi.nlm.nih.gov | |
Description | Data deposited in or computed by PubChem | |
Canonical SMILES |
CCCCC(=O)CCCCCCC(=O)OCC |
Source
|
Source | PubChem | |
URL | https://pubchem.ncbi.nlm.nih.gov | |
Description | Data deposited in or computed by PubChem | |
Molecular Formula |
C14H26O3 |
Source
|
Source | PubChem | |
URL | https://pubchem.ncbi.nlm.nih.gov | |
Description | Data deposited in or computed by PubChem | |
DSSTOX Substance ID |
DTXSID80539348 |
Source
|
Record name | Ethyl 8-oxododecanoate | |
Source | EPA DSSTox | |
URL | https://comptox.epa.gov/dashboard/DTXSID80539348 | |
Description | DSSTox provides a high quality public chemistry resource for supporting improved predictive toxicology. | |
Molecular Weight |
242.35 g/mol |
Source
|
Source | PubChem | |
URL | https://pubchem.ncbi.nlm.nih.gov | |
Description | Data deposited in or computed by PubChem | |
CAS No. |
97037-83-7 |
Source
|
Record name | Ethyl 8-oxododecanoate | |
Source | EPA DSSTox | |
URL | https://comptox.epa.gov/dashboard/DTXSID80539348 | |
Description | DSSTox provides a high quality public chemistry resource for supporting improved predictive toxicology. | |
Disclaimer and Information on In-Vitro Research Products
Please be aware that all articles and product information presented on BenchChem are intended solely for informational purposes. The products available for purchase on BenchChem are specifically designed for in-vitro studies, which are conducted outside of living organisms. In-vitro studies, derived from the Latin term "in glass," involve experiments performed in controlled laboratory settings using cells or tissues. It is important to note that these products are not categorized as medicines or drugs, and they have not received approval from the FDA for the prevention, treatment, or cure of any medical condition, ailment, or disease. We must emphasize that any form of bodily introduction of these products into humans or animals is strictly prohibited by law. It is essential to adhere to these guidelines to ensure compliance with legal and ethical standards in research and experimentation.