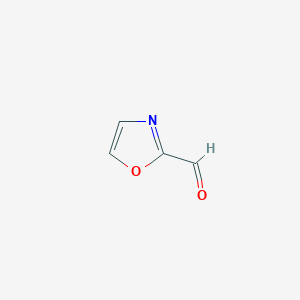
Oxazole-2-carbaldehyde
Overview
Description
Oxazole-2-carbaldehyde (CAS: 65373-52-6) is a five-membered heterocyclic compound with the molecular formula C₄H₃NO₂ and a molecular weight of 97.07 g/mol. It features an aldehyde functional group at the 2-position of the oxazole ring, making it a versatile building block in medicinal chemistry and organic synthesis. This compound is commonly utilized in the preparation of bioactive molecules, such as the intermediates 1-(Benzo[d]oxazol-2-yl)but-2-yn-1-ol (84% yield) and other derivatives for drug discovery . Its purity is typically listed as 97% in commercial catalogs, with suppliers like BLDpharm and Crysdot offering quantities ranging from 100 mg to 1 g .
Preparation Methods
Cyclization of α-Halo Ketones
One classical approach to synthesize oxazole-2-carbaldehyde involves the cyclization of α-halo ketones with suitable nitrogen sources. This method typically proceeds via nucleophilic substitution followed by ring closure to form the oxazole ring bearing the aldehyde group at position 2.
- Reaction Conditions: The α-halo ketone is reacted with an amide or related nitrogen nucleophile under reflux conditions, often in polar solvents.
- Advantages: Straightforward and direct formation of the oxazole ring.
- Limitations: Requires careful control of reaction conditions to avoid side reactions and ensure high purity.
Van Leusen Oxazole Synthesis
The Van Leusen synthesis is a widely used and versatile method for preparing oxazole derivatives, including this compound. It involves the reaction of aldehydes with tosylmethyl isocyanide (TosMIC) in the presence of a base.
- Mechanism: The aldehyde reacts with TosMIC under basic conditions (commonly potassium carbonate) in refluxing methanol or other solvents, leading to cyclization and formation of the oxazole ring.
- Reaction Conditions: Typically performed in methanol with K2CO3 at reflux temperature.
- Yields: Generally high, with good substrate scope.
- Green Chemistry Advances: Recent developments include the use of water as a solvent with β-cyclodextrin as a catalyst, allowing milder conditions and reduced environmental impact.
- Applications: This method is favored for its operational simplicity and broad applicability to various aldehyde substrates.
Oxidation of 5-Methyl-1,3-oxazole
For substituted oxazole-2-carbaldehydes, such as 5-methyl-1,3-oxazole-2-carbaldehyde, oxidation of the corresponding methyl-substituted oxazole is a common route.
- Oxidizing Agents: Potassium permanganate, chromium trioxide, or other strong oxidants.
- Reaction Conditions: Controlled oxidation to convert the methyl group at position 5 to an aldehyde at position 2.
- Industrial Relevance: This method is scalable and used in industrial settings for large-scale production.
- Challenges: Requires careful control to prevent over-oxidation or ring degradation.
Cyclization of 2-Amino-3-methylbut-2-enal
Another synthetic route involves acid-catalyzed cyclization of 2-amino-3-methylbut-2-enal.
- Reaction Conditions: Acid catalysts such as acetic acid or mineral acids promote cyclization.
- Outcome: Formation of 5-methyl-1,3-oxazole-2-carbaldehyde.
- Optimization: Reaction time, temperature, and catalyst concentration are critical for maximizing yield.
Van Leusen Synthesis Optimization: Recent studies have demonstrated that using catalytic amounts of base and water as a solvent with β-cyclodextrin significantly improves the environmental profile of the reaction while maintaining excellent yields (above 85%) at lower temperatures (~50 °C).
Oxidation Method Control: Industrial processes optimize oxidant concentration and reaction time to maximize aldehyde formation while minimizing ring cleavage. Monitoring by HPLC and TLC is standard to ensure product purity.
Cyclization Reactions: The Bischler-Napieralski type cyclization for oxazole derivatives involves refluxing aldehyde precursors with amines in acetic acid for 3–5 hours. Adjusting stoichiometry and temperature can increase yields up to 60%.
Structural Confirmation: X-ray crystallography and spectroscopic methods (NMR, IR, HRMS) are routinely used to confirm the structure and purity of this compound synthesized by these methods.
The preparation of this compound is achieved through several well-established synthetic routes:
- The Van Leusen oxazole synthesis stands out for its versatility, high yield, and adaptability to green chemistry principles.
- Oxidation of methyl-substituted oxazoles is industrially relevant for substituted derivatives.
- Cyclization of α-halo ketones and amino-enal precursors provide alternative routes with moderate yields.
- Optimization of reaction conditions, including solvent choice, temperature, and catalyst loading, is critical for maximizing yield and purity.
- Advanced analytical techniques ensure the structural integrity of the synthesized compound.
This comprehensive understanding of preparation methods supports the efficient synthesis of this compound for research and industrial applications.
Chemical Reactions Analysis
Types of Reactions: Oxazole-2-carbaldehyde undergoes various chemical reactions, including:
Oxidation: The aldehyde group can be oxidized to form the corresponding carboxylic acid.
Reduction: The aldehyde group can be reduced to form the corresponding alcohol.
Substitution: Electrophilic aromatic substitution can occur at the C5 position of the oxazole ring.
Common Reagents and Conditions:
Oxidation: Common oxidizing agents include potassium permanganate and chromium trioxide.
Reduction: Reducing agents such as sodium borohydride or lithium aluminum hydride are typically used.
Substitution: Electrophilic substitution reactions often require the presence of electron-donating groups to activate the ring.
Major Products:
Oxidation: Oxazole-2-carboxylic acid.
Reduction: Oxazole-2-methanol.
Substitution: Various substituted oxazole derivatives depending on the electrophile used.
Scientific Research Applications
Synthetic Routes
Several synthetic methods have been developed for the preparation of oxazole derivatives, including:
- Van Leusen Oxazole Synthesis : A classic method that involves the reaction of aldehydes with tosylmethyl isocyanide to form oxazoles.
- Cycloaddition Reactions : Recent studies have explored umpolung strategies for intermolecular cycloadditions, yielding various substituted oxazoles with high efficiency .
- Condensation Reactions : Oxazole-2-carbaldehyde can be synthesized through condensation reactions involving substituted acetophenones and urea or thiourea .
Biological Activities
This compound and its derivatives exhibit a wide range of biological activities, making them promising candidates for drug development.
Antimicrobial Activity
Research has demonstrated that oxazole derivatives possess significant antimicrobial properties. For instance, studies have shown that certain oxazole compounds can inhibit the growth of pathogens such as E. coli and Staphylococcus aureus, with minimum inhibitory concentrations (MICs) as low as 50 µg/mL.
Anticancer Properties
Oxazole derivatives have been investigated for their anticancer potential. They have been found to inhibit cancer cell proliferation by targeting specific enzymes involved in tumor growth. The mechanism often involves binding to active sites on enzymes or receptors, leading to the modulation of critical biological pathways.
Other Therapeutic Applications
The oxazole scaffold has been explored for various therapeutic applications, including:
- Anti-inflammatory agents
- Antiviral drugs
- Antitubercular compounds
Several oxazole-based compounds are currently undergoing clinical trials for their efficacy against diseases such as tuberculosis and cancer .
Case Study 1: Antimicrobial Efficacy
A study assessed the antimicrobial activity of this compound against common pathogens. The results indicated that this compound effectively inhibited bacterial growth, suggesting its potential use in developing new antimicrobial agents.
Case Study 2: Anticancer Activity
In another investigation, a series of oxazole derivatives were synthesized and evaluated for their anticancer properties. Some compounds exhibited potent activity against various cancer cell lines, demonstrating the therapeutic potential of this chemical class.
Mechanism of Action
The mechanism of action of oxazole-2-carbaldehyde and its derivatives often involves interactions with biological targets such as enzymes and receptors. The heterocyclic ring structure allows for binding to active sites, leading to inhibition or activation of specific biological pathways. For example, some oxazole derivatives have been shown to inhibit enzymes involved in cancer cell proliferation .
Comparison with Similar Compounds
Comparison with Structurally Similar Compounds
Key Structural and Functional Differences
The following table compares Oxazole-2-carbaldehyde with analogous heterocyclic aldehydes, highlighting substituent effects and applications:
Physicochemical and Reactivity Profiles
- Electronic Effects: Fluorine and bromine substituents (e.g., in 5-Fluorobenzo[d]this compound and 4-Bromobenzo[d]this compound) increase electrophilicity at the aldehyde group, enhancing reactivity in nucleophilic additions or metal-catalyzed cross-couplings .
Solubility and Stability :
Biological Activity
Oxazole-2-carbaldehyde is a heterocyclic compound that has garnered significant interest in medicinal chemistry due to its diverse biological activities. This article explores the biological activity of this compound, focusing on its antimicrobial, anticancer, and antiviral properties, alongside relevant synthetic pathways and structure-activity relationships (SAR).
Chemical Structure and Properties
This compound features a five-membered ring containing one nitrogen and one oxygen atom, with an aldehyde functional group attached. This unique structure allows for various chemical reactions and interactions with biological targets, enhancing its potential as a therapeutic agent.
Antimicrobial Activity
Numerous studies have demonstrated the antimicrobial properties of this compound and its derivatives. These compounds have shown effectiveness against various bacterial and fungal strains.
Table 1: Antimicrobial Activity of Oxazole Derivatives
Compound | MIC (µg/ml) against Bacteria/Fungi |
---|---|
11 | Candida albicans: 1.6 |
Candida tropicalis: 3.2 | |
Aspergillus niger: 1.6 | |
12 | Candida albicans: 0.8 |
Aspergillus flavus: 1.6 | |
Reference (5-Fluorocytosine) | Candida albicans: 3.2 |
The above table summarizes the minimum inhibitory concentrations (MICs) for selected oxazole derivatives against various pathogens, indicating their potential as antimicrobial agents .
Anticancer Properties
This compound has also been investigated for its anticancer activity. Research indicates that certain oxazole derivatives can inhibit cancer cell proliferation through mechanisms involving enzyme inhibition and receptor interaction.
Case Study: Inhibition of Cancer Cell Proliferation
One study focused on the synthesis of oxazole derivatives that demonstrated significant cytotoxic effects against human cancer cell lines. The mechanism was attributed to the compounds' ability to inhibit specific enzymes involved in tumor growth, showcasing their potential as anticancer therapeutics .
Antiviral Activity
Recent investigations have highlighted the antiviral potential of oxazole-based compounds, particularly against viruses such as SARS-CoV-2. A family of synthetic oxazole-based macrocycles exhibited significant activity against this virus, suggesting a promising avenue for developing new antiviral agents.
Table 2: Antiviral Activity Against SARS-CoV-2
Compound | Activity Level |
---|---|
Macrocycle A | Significant Inhibition |
Macrocycle B | Moderate Inhibition |
These findings underscore the relevance of oxazole derivatives in combating viral infections, particularly in light of global health challenges posed by emerging viruses .
Structure-Activity Relationship (SAR)
Understanding the SAR of oxazole derivatives is crucial for optimizing their biological activities. The presence of specific substituents on the oxazole ring significantly influences their pharmacological properties.
Key Findings from SAR Studies:
- Substituents at the C-4 position enhance antimicrobial activity.
- Electron-withdrawing groups at the C-5 position improve anticancer efficacy.
- Modifications to the aldehyde group can alter binding affinity to biological targets.
The versatility of the oxazole moiety makes it a valuable scaffold in drug design, facilitating the development of compounds with tailored biological activities .
Synthetic Routes
The synthesis of this compound can be achieved through several methods, including:
- Van Leusen Reaction : A widely used method involving the reaction of aldehydes with TosMIC to produce oxazoles.
- N-Acylation Reactions : Acylation at the nitrogen position can yield various substituted oxazoles with enhanced biological activities.
These synthetic approaches allow for the generation of a diverse library of oxazole derivatives suitable for biological testing .
Q & A
Basic Research Questions
Q. What are the established synthetic routes for Oxazole-2-carbaldehyde, and how do their yields and purity compare?
- Methodology : Common methods include formylation of oxazole derivatives using Vilsmeier-Haack or Duff reactions. For example, benzo[d]this compound synthesis achieved 84% yield via alkyne addition (). Characterization via ESIMS (electrospray ionization mass spectrometry) and purity assessment (e.g., 97% purity per ) are critical. Researchers should validate yields using HPLC or GC-MS and cross-reference synthetic protocols with peer-reviewed literature.
Q. Which spectroscopic techniques are most effective for characterizing this compound, and how should data be interpreted?
- Methodology : Nuclear Magnetic Resonance (NMR) is standard for structural elucidation, particularly - and -NMR. Infrared (IR) spectroscopy identifies functional groups like the aldehyde moiety. Mass spectrometry (e.g., ESIMS m/z 188.2 in ) confirms molecular weight. Researchers must account for solvent effects and calibration errors when comparing spectral data across studies .
Q. What safety precautions are essential when handling this compound in laboratory settings?
- Methodology : Follow protocols for skin/eye protection and ventilation, as the compound may pose acute toxicity risks (). Safety Data Sheets (SDS) recommend consulting physicians if exposed and storing the compound away from incompatible materials (e.g., strong oxidizers). Stability under recommended storage conditions (room temperature, inert atmosphere) should be verified experimentally .
Advanced Research Questions
Q. How can reaction conditions be optimized to enhance the yield of this compound in multi-step syntheses?
- Methodology : Systematic variation of catalysts (e.g., Lewis acids), solvents (polar vs. non-polar), and temperature gradients can improve efficiency. For instance, highlights yield differences (84–94%) depending on the aldehyde substrate. Design of Experiments (DoE) or response surface methodology (RSM) can statistically identify optimal conditions while minimizing side reactions .
Q. What strategies resolve contradictions in reported NMR chemical shifts for this compound across studies?
- Methodology : Discrepancies may arise from solvent effects (e.g., DMSO vs. CDCl), impurities, or spectrometer calibration. Researchers should replicate experiments under standardized conditions and compare results with computational predictions (e.g., density functional theory (DFT)-calculated shifts). Cross-validation using 2D NMR (COSY, HSQC) can clarify ambiguous assignments .
Q. How do computational methods like DFT contribute to understanding the electronic properties of this compound?
- Methodology : DFT calculations model molecular orbitals, charge distribution, and reactivity sites (e.g., aldehyde group electrophilicity). Such analyses predict regioselectivity in reactions, such as nucleophilic additions. Researchers should validate computational results with experimental data (e.g., X-ray crystallography or kinetic studies) .
Q. What mechanistic insights explain this compound’s role in heterocyclic ring-forming reactions?
- Methodology : Isotopic labeling (e.g., -aldehyde) tracks carbon migration in cycloadditions. Kinetic studies (e.g., variable-temperature NMR) reveal rate-determining steps. Combining experimental data with computational transition-state analysis (e.g., NEB calculations) provides a holistic mechanistic understanding .
Q. How does this compound’s stability vary under non-standard storage conditions, and what decomposition products form?
- Methodology : Accelerated stability studies (e.g., high humidity, elevated temperature) coupled with LC-MS monitor degradation. notes potential hazardous decomposition products, which can be identified via fragmentation patterns. Control experiments under inert atmospheres (N, Ar) assess oxidative stability .
Q. Data Analysis and Reproducibility
Q. What frameworks ensure reproducibility in synthetic protocols for this compound?
- Methodology : Detailed documentation of reagent purity (e.g., ≥97% per ), solvent batch numbers, and equipment calibration (e.g., NMR spectrometer frequency) is critical. Open-source platforms like protocols.io encourage transparency. Independent replication studies and inter-lab comparisons mitigate procedural biases .
Q. How should researchers address contradictory bioactivity data for this compound derivatives in pharmacological studies?
- Methodology : Meta-analyses of structure-activity relationships (SAR) across studies can identify confounding variables (e.g., assay type, cell line variability). Dose-response curves and positive/negative controls validate activity. Collaborations with independent labs enhance data robustness .
Q. Ethical and Methodological Considerations
Q. What ethical guidelines apply when incorporating this compound in biomedical research involving human subjects?
- Methodology : Adhere to Institutional Review Board (IRB) protocols for toxicity and informed consent (). Preclinical safety profiles (e.g., acute toxicity in ) must precede human trials. Transparent reporting of adverse events ensures compliance with ICMJE standards .
Q. How can mixed-methods approaches enhance the interpretation of this compound’s reactivity data?
Properties
IUPAC Name |
1,3-oxazole-2-carbaldehyde | |
---|---|---|
Source | PubChem | |
URL | https://pubchem.ncbi.nlm.nih.gov | |
Description | Data deposited in or computed by PubChem | |
InChI |
InChI=1S/C4H3NO2/c6-3-4-5-1-2-7-4/h1-3H | |
Source | PubChem | |
URL | https://pubchem.ncbi.nlm.nih.gov | |
Description | Data deposited in or computed by PubChem | |
InChI Key |
TYHOSUCCUICRLM-UHFFFAOYSA-N | |
Source | PubChem | |
URL | https://pubchem.ncbi.nlm.nih.gov | |
Description | Data deposited in or computed by PubChem | |
Canonical SMILES |
C1=COC(=N1)C=O | |
Source | PubChem | |
URL | https://pubchem.ncbi.nlm.nih.gov | |
Description | Data deposited in or computed by PubChem | |
Molecular Formula |
C4H3NO2 | |
Source | PubChem | |
URL | https://pubchem.ncbi.nlm.nih.gov | |
Description | Data deposited in or computed by PubChem | |
DSSTOX Substance ID |
DTXSID20564013 | |
Record name | 1,3-Oxazole-2-carbaldehyde | |
Source | EPA DSSTox | |
URL | https://comptox.epa.gov/dashboard/DTXSID20564013 | |
Description | DSSTox provides a high quality public chemistry resource for supporting improved predictive toxicology. | |
Molecular Weight |
97.07 g/mol | |
Source | PubChem | |
URL | https://pubchem.ncbi.nlm.nih.gov | |
Description | Data deposited in or computed by PubChem | |
CAS No. |
65373-52-6 | |
Record name | 1,3-Oxazole-2-carbaldehyde | |
Source | EPA DSSTox | |
URL | https://comptox.epa.gov/dashboard/DTXSID20564013 | |
Description | DSSTox provides a high quality public chemistry resource for supporting improved predictive toxicology. | |
Record name | 1,3-Oxazole-2-carboxaldehyde | |
Source | European Chemicals Agency (ECHA) | |
URL | https://echa.europa.eu/information-on-chemicals | |
Description | The European Chemicals Agency (ECHA) is an agency of the European Union which is the driving force among regulatory authorities in implementing the EU's groundbreaking chemicals legislation for the benefit of human health and the environment as well as for innovation and competitiveness. | |
Explanation | Use of the information, documents and data from the ECHA website is subject to the terms and conditions of this Legal Notice, and subject to other binding limitations provided for under applicable law, the information, documents and data made available on the ECHA website may be reproduced, distributed and/or used, totally or in part, for non-commercial purposes provided that ECHA is acknowledged as the source: "Source: European Chemicals Agency, http://echa.europa.eu/". Such acknowledgement must be included in each copy of the material. ECHA permits and encourages organisations and individuals to create links to the ECHA website under the following cumulative conditions: Links can only be made to webpages that provide a link to the Legal Notice page. | |
Retrosynthesis Analysis
AI-Powered Synthesis Planning: Our tool employs the Template_relevance Pistachio, Template_relevance Bkms_metabolic, Template_relevance Pistachio_ringbreaker, Template_relevance Reaxys, Template_relevance Reaxys_biocatalysis model, leveraging a vast database of chemical reactions to predict feasible synthetic routes.
One-Step Synthesis Focus: Specifically designed for one-step synthesis, it provides concise and direct routes for your target compounds, streamlining the synthesis process.
Accurate Predictions: Utilizing the extensive PISTACHIO, BKMS_METABOLIC, PISTACHIO_RINGBREAKER, REAXYS, REAXYS_BIOCATALYSIS database, our tool offers high-accuracy predictions, reflecting the latest in chemical research and data.
Strategy Settings
Precursor scoring | Relevance Heuristic |
---|---|
Min. plausibility | 0.01 |
Model | Template_relevance |
Template Set | Pistachio/Bkms_metabolic/Pistachio_ringbreaker/Reaxys/Reaxys_biocatalysis |
Top-N result to add to graph | 6 |
Feasible Synthetic Routes
Disclaimer and Information on In-Vitro Research Products
Please be aware that all articles and product information presented on BenchChem are intended solely for informational purposes. The products available for purchase on BenchChem are specifically designed for in-vitro studies, which are conducted outside of living organisms. In-vitro studies, derived from the Latin term "in glass," involve experiments performed in controlled laboratory settings using cells or tissues. It is important to note that these products are not categorized as medicines or drugs, and they have not received approval from the FDA for the prevention, treatment, or cure of any medical condition, ailment, or disease. We must emphasize that any form of bodily introduction of these products into humans or animals is strictly prohibited by law. It is essential to adhere to these guidelines to ensure compliance with legal and ethical standards in research and experimentation.