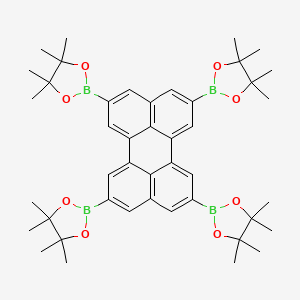
2,5,8,11-Tetrakis(4,4,5,5-tetramethyl-1,3,2-dioxaborolan-2-yl)perylene
- Click on QUICK INQUIRY to receive a quote from our team of experts.
- With the quality product at a COMPETITIVE price, you can focus more on your research.
Overview
Description
2,5,8,11-Tetrakis(4,4,5,5-tetramethyl-1,3,2-dioxaborolan-2-yl)perylene is a complex organic compound with the molecular formula C44H56B4O8 It is known for its unique structure, which includes four boronate ester groups attached to a perylene core
Preparation Methods
Synthetic Routes and Reaction Conditions
The synthesis of 2,5,8,11-Tetrakis(4,4,5,5-tetramethyl-1,3,2-dioxaborolan-2-yl)perylene typically involves the borylation of perylene derivatives. One common method is the iridium-catalyzed borylation of polycyclic aromatic hydrocarbons. The reaction conditions often include the use of iridium catalysts, such as [Ir(cod)Cl]2, and bis(pinacolato)diboron (B2Pin2) as the boron source .
Industrial Production Methods
While specific industrial production methods for this compound are not widely documented, the general approach would involve scaling up the laboratory synthesis methods. This would include optimizing the reaction conditions for larger-scale production, ensuring the availability of high-purity starting materials, and implementing efficient purification techniques to obtain the desired product.
Chemical Reactions Analysis
Types of Reactions
2,5,8,11-Tetrakis(4,4,5,5-tetramethyl-1,3,2-dioxaborolan-2-yl)perylene undergoes various chemical reactions, including:
Substitution: Reactions where one functional group is replaced by another.
Coupling Reactions: Formation of carbon-carbon bonds through reactions such as Suzuki-Miyaura coupling.
Common Reagents and Conditions
Iridium Catalysts: Used in borylation reactions.
Bis(pinacolato)diboron (B2Pin2): A common boron source.
Palladium Catalysts: Used in coupling reactions like Suzuki-Miyaura coupling.
Major Products
The major products formed from these reactions include various boronate esters and coupled products, which can be further utilized in the synthesis of more complex organic materials .
Scientific Research Applications
2,5,8,11-Tetrakis(4,4,5,5-tetramethyl-1,3,2-dioxaborolan-2-yl)perylene has several scientific research applications:
Organic Electronics: Used as a building block for organic semiconductors and light-emitting diodes (LEDs).
Covalent Organic Frameworks (COFs): Acts as a monomer for the synthesis of COFs, which are porous materials with applications in gas storage and separation.
Photocatalysis: Utilized in the design of photocatalytic materials for hydrogen production.
Sensors: Employed in the development of chemical sensors due to its unique electronic properties.
Mechanism of Action
The mechanism by which 2,5,8,11-Tetrakis(4,4,5,5-tetramethyl-1,3,2-dioxaborolan-2-yl)perylene exerts its effects is primarily through its electronic structure. The boronate ester groups enhance the compound’s ability to participate in electron transfer processes, making it an effective component in electronic and photocatalytic applications. The perylene core provides a stable and conjugated system that facilitates these processes .
Comparison with Similar Compounds
Similar Compounds
Perylene-2,5,8,11-tetracarboxylic dianhydride: Another perylene derivative used in organic electronics.
Perylene-3,4,9,10-tetracarboxylic dianhydride: Known for its applications in pigments and dyes.
Perylene-2,5,8,11-tetrakis(4-carboxyphenyl): Used in the synthesis of COFs and other advanced materials.
Uniqueness
2,5,8,11-Tetrakis(4,4,5,5-tetramethyl-1,3,2-dioxaborolan-2-yl)perylene is unique due to the presence of four boronate ester groups, which significantly enhance its reactivity and versatility in various chemical reactions. This makes it a valuable compound for the development of new materials with advanced electronic and catalytic properties .
Properties
Molecular Formula |
C44H56B4O8 |
---|---|
Molecular Weight |
756.2 g/mol |
IUPAC Name |
4,4,5,5-tetramethyl-2-[5,8,11-tris(4,4,5,5-tetramethyl-1,3,2-dioxaborolan-2-yl)perylen-2-yl]-1,3,2-dioxaborolane |
InChI |
InChI=1S/C44H56B4O8/c1-37(2)38(3,4)50-45(49-37)27-17-25-18-28(46-51-39(5,6)40(7,8)52-46)23-33-34-24-30(48-55-43(13,14)44(15,16)56-48)20-26-19-29(47-53-41(9,10)42(11,12)54-47)22-32(36(26)34)31(21-27)35(25)33/h17-24H,1-16H3 |
InChI Key |
FIBSLDZCCRAMCO-UHFFFAOYSA-N |
Canonical SMILES |
B1(OC(C(O1)(C)C)(C)C)C2=CC3=C4C(=C2)C5=CC(=CC6=C5C(=CC(=C6)B7OC(C(O7)(C)C)(C)C)C4=CC(=C3)B8OC(C(O8)(C)C)(C)C)B9OC(C(O9)(C)C)(C)C |
Origin of Product |
United States |
Disclaimer and Information on In-Vitro Research Products
Please be aware that all articles and product information presented on BenchChem are intended solely for informational purposes. The products available for purchase on BenchChem are specifically designed for in-vitro studies, which are conducted outside of living organisms. In-vitro studies, derived from the Latin term "in glass," involve experiments performed in controlled laboratory settings using cells or tissues. It is important to note that these products are not categorized as medicines or drugs, and they have not received approval from the FDA for the prevention, treatment, or cure of any medical condition, ailment, or disease. We must emphasize that any form of bodily introduction of these products into humans or animals is strictly prohibited by law. It is essential to adhere to these guidelines to ensure compliance with legal and ethical standards in research and experimentation.