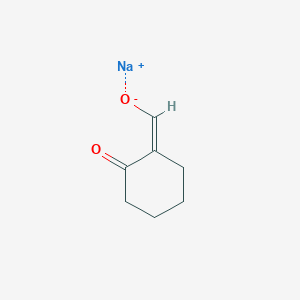
Sodium (2-oxocyclohexylidene)methanolate
- Click on QUICK INQUIRY to receive a quote from our team of experts.
- With the quality product at a COMPETITIVE price, you can focus more on your research.
Overview
Description
Sodium (2-oxocyclohexylidene)methanolate is a chemical compound with the molecular formula C7H9NaO2 It is a sodium salt derivative of a cyclohexanone compound, characterized by the presence of a methanolate group
Preparation Methods
Synthetic Routes and Reaction Conditions: Sodium (2-oxocyclohexylidene)methanolate can be synthesized through the treatment of 3-methylcyclohexanone with ethyl formate in the presence of sodium methoxide . This reaction involves the formation of the sodium salt of the intermediate product, which is then isolated and purified.
Industrial Production Methods: While specific industrial production methods for this compound are not extensively documented, the general approach involves large-scale synthesis using the aforementioned reaction conditions. The process is optimized for yield and purity, ensuring the compound meets the required standards for research and industrial applications.
Chemical Reactions Analysis
Types of Reactions: Sodium (2-oxocyclohexylidene)methanolate undergoes various chemical reactions, including:
Oxidation: The compound can be oxidized to form corresponding ketones or carboxylic acids.
Reduction: Reduction reactions can convert it into alcohols or other reduced forms.
Substitution: It can participate in nucleophilic substitution reactions, where the methanolate group is replaced by other nucleophiles.
Common Reagents and Conditions:
Oxidation: Common oxidizing agents include potassium permanganate (KMnO4) and chromium trioxide (CrO3).
Reduction: Reducing agents such as lithium aluminum hydride (LiAlH4) and sodium borohydride (NaBH4) are typically used.
Substitution: Nucleophiles like amines, thiols, and halides can be used under appropriate conditions.
Major Products Formed: The major products formed from these reactions depend on the specific reagents and conditions used. For example, oxidation may yield carboxylic acids, while reduction can produce alcohols.
Scientific Research Applications
Sodium (2-oxocyclohexylidene)methanolate has several applications in scientific research, including:
Chemistry: It is used as an intermediate in the synthesis of various organic compounds, including heterocycles and pharmaceuticals.
Biology: The compound’s derivatives are studied for their potential biological activities, such as antimicrobial and anticancer properties.
Medicine: Research into its derivatives explores potential therapeutic applications, including drug development.
Industry: It is used in the production of fine chemicals and as a reagent in various industrial processes.
Mechanism of Action
The mechanism by which sodium (2-oxocyclohexylidene)methanolate exerts its effects involves its reactivity as a nucleophile and its ability to participate in various chemical reactions. The compound can interact with molecular targets through nucleophilic attack, leading to the formation of new chemical bonds and the generation of biologically active compounds. The specific pathways involved depend on the nature of the reactions and the target molecules.
Comparison with Similar Compounds
Sodium (2-methyl-6-oxocyclohexylidene)methanolate: This compound is similar in structure but has a methyl group at the 2-position.
Sodium (2-oxocyclopentylidene)methanolate: A related compound with a cyclopentane ring instead of a cyclohexane ring.
Sodium (2-oxocycloheptylidene)methanolate: This compound features a cycloheptane ring.
Uniqueness: Sodium (2-oxocyclohexylidene)methanolate is unique due to its specific ring size and the presence of the methanolate group, which imparts distinct chemical properties and reactivity. Its ability to form various derivatives and participate in diverse chemical reactions makes it a valuable compound in research and industrial applications.
Biological Activity
Sodium (2-oxocyclohexylidene)methanolate is a compound of interest due to its potential biological activities, particularly in the context of cancer treatment. This article explores its synthesis, biological properties, and relevant case studies, supported by data tables and research findings.
Synthesis and Characterization
This compound can be synthesized through various chemical reactions involving cyclohexanone derivatives. For example, the reaction of 3-methylcyclohexanone with ethyl formate in the presence of sodium methoxide yields this compound . The characterization of synthesized compounds typically involves techniques such as infrared spectroscopy, NMR, and elemental analysis to confirm their structures .
Anticancer Properties
Research has demonstrated that this compound exhibits significant anticancer activity. A study involving several derivatives indicated that compounds derived from this compound showed similar growth inhibition profiles to their cyclohexanone counterparts, with notable efficacy against various tumor cell lines. The following table summarizes the growth inhibition metrics for selected derivatives:
Compound | Tumor Cell Line | GI50 (nM) | LC50 (nM) |
---|---|---|---|
6b | MDA-MB-435 (melanoma) | 100 | 925 |
9a | MDA-MB-468 (breast) | 70 | 800 |
12 | SF-295 (CNS) | 150 | 600 |
The most active derivative, identified as 9a , exhibited a GI50 of 70 nM against the MDA-MB-435 cell line, indicating its potency in inhibiting cancer cell growth . Furthermore, derivative 12 was tested in a mouse xenograft model, demonstrating partial inhibition of tumor growth despite its poor solubility .
The mechanism by which this compound exerts its biological effects is an area of active research. Studies have indicated that it may inhibit specific enzymes involved in DNA repair, such as tyrosyl-DNA phosphodiesterase I (TDP1), with derivative 9d showing an IC50 value of approximately 0.5 μM against this target . The lack of significant affinity towards a broad range of kinases suggests a selective mode of action that warrants further investigation.
Case Studies and Research Findings
Several studies have focused on the biological evaluation of this compound derivatives:
- In Vitro Studies : Derivatives were screened against the NCI60 tumor cell panel, revealing that modifications to the cycloaliphatic moiety can enhance anticancer activity. The results indicated that larger aliphatic ring systems are generally more favorable for biological activity .
- In Vivo Studies : In a mouse model, derivative 12 was administered intraperitoneally, resulting in tumor size reduction; however, statistical significance was not achieved. This highlights the need for improved formulations to enhance solubility and bioavailability .
- Structure-Activity Relationship (SAR) : The SAR analysis revealed that ortho/meta substitution patterns on the phenyl ring significantly influence anticancer efficacy. Compounds with specific substitutions demonstrated enhanced growth inhibition compared to their unsubstituted counterparts .
Properties
Molecular Formula |
C7H9NaO2 |
---|---|
Molecular Weight |
148.13 g/mol |
IUPAC Name |
sodium;(Z)-(2-oxocyclohexylidene)methanolate |
InChI |
InChI=1S/C7H10O2.Na/c8-5-6-3-1-2-4-7(6)9;/h5,8H,1-4H2;/q;+1/p-1/b6-5-; |
InChI Key |
KUDIADBKFNARIF-YSMBQZINSA-M |
Isomeric SMILES |
C1CCC(=O)/C(=C\[O-])/C1.[Na+] |
Canonical SMILES |
C1CCC(=O)C(=C[O-])C1.[Na+] |
Origin of Product |
United States |
Disclaimer and Information on In-Vitro Research Products
Please be aware that all articles and product information presented on BenchChem are intended solely for informational purposes. The products available for purchase on BenchChem are specifically designed for in-vitro studies, which are conducted outside of living organisms. In-vitro studies, derived from the Latin term "in glass," involve experiments performed in controlled laboratory settings using cells or tissues. It is important to note that these products are not categorized as medicines or drugs, and they have not received approval from the FDA for the prevention, treatment, or cure of any medical condition, ailment, or disease. We must emphasize that any form of bodily introduction of these products into humans or animals is strictly prohibited by law. It is essential to adhere to these guidelines to ensure compliance with legal and ethical standards in research and experimentation.