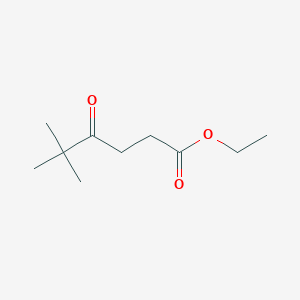
Ethyl 5,5-dimethyl-4-oxohexanoate
Overview
Description
Its structure features a 4-oxohexanoate backbone substituted with two methyl groups at the C5 position and an ethyl ester group at the terminal carboxylate. This compound is primarily synthesized via the reaction of β-keto esters (e.g., Methyl 4,4-dimethyl-3-oxopentanoate) with diethylzinc and methylene iodide under inert conditions, a method optimized for high yields (~80%) and scalability . As a keto ester, it serves as a versatile intermediate in organic synthesis, particularly in the preparation of heterocycles and bioactive molecules .
Preparation Methods
Preparation Methods of Ethyl 5,5-dimethyl-4-oxohexanoate
Chain Extension of β-Keto Esters Using Diethylzinc Mediator
One of the most documented and efficient synthetic routes involves the chain extension of a β-keto ester such as methyl 4,4-dimethyl-3-oxopentanoate. This method uses diethylzinc as a mediator and methylene iodide as a chain-extending agent under inert atmosphere conditions.
- Dissolve methyl 4,4-dimethyl-3-oxopentanoate in methylene chloride.
- Add diethylzinc slowly under nitrogen atmosphere to avoid oxidation.
- Introduce methylene iodide dropwise to the reaction mixture.
- Stir the mixture to allow chain extension.
- Quench the reaction with saturated aqueous ammonium chloride.
- Extract the organic layer and purify the product by vacuum distillation.
This method yields this compound as a clear, colorless oil with high purity and good yield (typically 89–94%).
Step | Reagents/Conditions | Purpose |
---|---|---|
Dissolution | Methyl 4,4-dimethyl-3-oxopentanoate in CH2Cl2 | Solvent medium |
Addition | Diethylzinc under N2 atmosphere | Mediator for chain extension |
Dropwise addition | Methylene iodide | Chain extension agent |
Stirring | Room temperature | Reaction progression |
Quenching | Saturated NH4Cl aqueous solution | Terminate reaction |
Extraction and purification | Vacuum distillation | Isolate pure product |
Claisen Condensation and Alkylation of Keto Esters
Another common synthetic approach is based on Claisen condensation or direct alkylation of β-keto esters. This involves:
- Using methyl or ethyl esters of β-keto acids.
- Alkylation with methyl halides (e.g., methyl iodide) in the presence of strong bases such as sodium hydride (NaH) or lithium diisopropylamide (LDA).
- The reaction proceeds via C-alkylation under Friedel-Crafts-like conditions, where the keto ester acts as a nucleophile.
- Subsequent esterification with ethanol under acid catalysis (e.g., sulfuric acid) can be used to obtain the ethyl ester derivative.
This method requires careful control of reaction conditions to avoid over-alkylation and side reactions. Purity is typically confirmed by gas chromatography-mass spectrometry (GC-MS) and proton nuclear magnetic resonance (¹H NMR) spectroscopy.
Hydrolysis and Re-esterification Route
An alternative approach involves:
- Hydrolysis of the ester group to yield 5,5-dimethyl-4-oxohexanoic acid.
- Followed by esterification with ethanol under acidic conditions (e.g., H₂SO₄ catalyst).
- This two-step process allows for the preparation of the ethyl ester from the corresponding acid.
Hydrolysis can be performed under acidic or basic conditions, with yields ranging from 75% to 90%. Microwave-assisted hydrolysis has been reported to reduce reaction times significantly.
Detailed Research Findings and Analysis
Reaction Mechanism Insights
- The chain extension reaction mediated by diethylzinc involves nucleophilic attack on methylene iodide, forming an organozinc intermediate that reacts with the β-keto ester.
- The keto group’s electrophilicity and the steric hindrance from the 5,5-dimethyl substitution influence the reaction rate and yield.
- Alkylation reactions are sensitive to steric and electronic effects; the 5,5-dimethyl groups reduce reactivity compared to less substituted analogs.
Spectroscopic Characterization
- ¹H NMR: Ethyl ester protons appear as a triplet (~1.2 ppm, CH₃) and quartet (~4.1 ppm, CH₂). The dimethyl groups at the 5-position show singlets near 1.0–1.2 ppm. The keto group deshields adjacent protons, typically appearing as a singlet around 2.5 ppm.
- ¹³C NMR and DEPT-135: Confirm the presence of carbonyl carbons and methyl carbons.
- X-ray Crystallography: Used to confirm molecular geometry and substitution pattern, especially the steric effects of the 5,5-dimethyl groups.
Yield and Purity Data
Preparation Method | Yield (%) | Purification Method | Purity Confirmation Techniques |
---|---|---|---|
Chain extension with diethylzinc | 89–94 | Vacuum distillation | GC-MS, ¹H NMR |
Claisen condensation/alkylation | 60–85 | Recrystallization, HPLC | GC-MS, ¹H NMR |
Hydrolysis and re-esterification | 75–90 | Extraction, distillation | TLC, GC-MS |
Summary Table of Preparation Methods
Method | Key Reagents/Conditions | Advantages | Limitations |
---|---|---|---|
Chain extension (diethylzinc) | Methyl 4,4-dimethyl-3-oxopentanoate, diethylzinc, methylene iodide, CH2Cl2, N2 atmosphere | High yield, straightforward purification | Requires inert atmosphere, sensitive reagents |
Claisen condensation/alkylation | β-keto ester, methyl halide, strong base (NaH, LDA), acid catalysis for esterification | Versatile, well-studied | Steric hindrance reduces yield, side reactions possible |
Hydrolysis + esterification | Acid/base hydrolysis, ethanol, acid catalyst | Allows access from acid precursor | Multi-step, longer reaction times |
Chemical Reactions Analysis
Ethyl 5,5-dimethyl-4-oxohexanoate undergoes various chemical reactions, including:
Oxidation: The keto group can be oxidized to form carboxylic acids.
Reduction: The keto group can be reduced to form alcohols.
Substitution: The ester group can undergo nucleophilic substitution reactions to form different esters or acids.
Common reagents used in these reactions include oxidizing agents like potassium permanganate, reducing agents like sodium borohydride, and nucleophiles like alcohols and amines. The major products formed from these reactions depend on the specific conditions and reagents used .
Scientific Research Applications
Chemical Properties and Structure
Ethyl 5,5-dimethyl-4-oxohexanoate is classified as a β-ketoester with the molecular formula and a molecular weight of approximately 228.33 g/mol. Its structure features both ketone and ester functional groups, contributing to its reactivity and utility in synthetic chemistry.
Pharmaceutical Applications
1.1 Precursor for Bioactive Compounds
This compound serves as a precursor in the synthesis of various bioactive molecules. Its structural attributes allow for modifications that can lead to the development of new pharmaceuticals aimed at treating diverse medical conditions.
1.2 Antimicrobial Activity
Research has indicated that compounds derived from this compound exhibit antimicrobial properties. This makes it a candidate for further exploration in the development of antibiotics or antifungal agents.
Synthetic Applications
2.1 Organic Synthesis
The compound is utilized in organic synthesis as a building block for more complex molecules. Its ability to undergo various chemical reactions, such as condensation and acylation, makes it versatile in creating different organic compounds.
2.2 Reaction Mechanisms
The unique structure allows this compound to participate in multiple reaction pathways:
- Michael Addition Reactions : It can act as an electrophile in Michael addition reactions, facilitating the formation of carbon-carbon bonds.
- Cyclization Reactions : The compound can undergo cyclization to form cyclic structures, which are often important in medicinal chemistry.
Synthesis of Novel Compounds
A study published in Journal of Organic Chemistry demonstrated the successful use of this compound in synthesizing novel heterocyclic compounds with potential pharmacological activities. The research highlighted the compound's role as a versatile intermediate that can be modified to enhance biological activity.
Anticancer Research
In another case study, researchers investigated derivatives of this compound for their anticancer properties. The findings suggested that certain derivatives exhibited selective cytotoxicity against cancer cell lines while sparing normal cells, indicating potential for further development into anticancer therapies.
Comparative Analysis with Related Compounds
To understand the uniqueness of this compound, a comparative analysis with similar compounds is essential:
Compound Name | Structural Characteristics | Similarity Index |
---|---|---|
Ethyl 5,5-dimethyl-3-oxohexanoate | Lacks ketone at C4; simpler structure | 0.97 |
Butyl 4,4-dimethyl-3-oxopentanoate | Different alkyl chain; similar functional groups | 0.95 |
Ethyl 6-methyl-3-oxoheptanoate | Longer carbon chain; different methyl position | 0.94 |
This table illustrates how this compound stands out due to its specific arrangement of substituents and functional groups.
Mechanism of Action
The mechanism of action of ethyl 5,5-dimethyl-4-oxohexanoate involves its reactivity due to the presence of the keto and ester groups. These functional groups can participate in various chemical reactions, such as nucleophilic addition and substitution, which are fundamental in organic synthesis. The molecular targets and pathways involved depend on the specific reactions and applications being studied .
Comparison with Similar Compounds
Comparison with Structurally Similar Compounds
Ethyl 5-Oxohexanoate (CAS 13984-57-1)
Molecular Formula : C₈H₁₄O₃
Molecular Weight : 158.20 g/mol
Key Differences :
- Lacks the 5,5-dimethyl substitution present in the target compound, resulting in a simpler hexanoate backbone.
- Physical Properties: Lower molecular weight correlates with a lower boiling point (estimated 210–220°C) compared to Ethyl 5,5-dimethyl-4-oxohexanoate (~250°C) . Applications: Used in flavoring agents and as a precursor for γ-lactams .
Mthis compound (CAS 34553-32-7)
Molecular Formula : C₉H₁₆O₃
Molecular Weight : 172.22 g/mol
Key Differences :
- Methyl ester vs. ethyl ester group: The shorter alkyl chain reduces lipophilicity (logP ~1.2 vs. ~1.8 for the ethyl analogue), affecting solubility in polar solvents .
- Synthesis : Prepared via analogous methods but with methyl β-keto ester precursors. The reaction with diethylzinc and methylene iodide proceeds similarly but may require adjusted stoichiometry .
Applications : Intermediate in pharmaceutical syntheses, particularly for anti-inflammatory agents .
Ethyl 4-Acetyl-5-oxohexanoate (CAS 2832102)
Molecular Formula : C₁₀H₁₆O₄
Molecular Weight : 200.23 g/mol
Key Differences :
- Features dual carbonyl groups (4-acetyl and 5-oxo) compared to the single 4-oxo group in the target compound.
- The additional acetyl group enhances electrophilicity, making it more reactive in Michael additions or aldol condensations .
Applications : Used in tandem mass spectrometry studies due to its fragmentation patterns .
Ethyl 5,6-Epoxy-4-oxohexanoate
Molecular Formula : C₈H₁₂O₄
Molecular Weight : 172.18 g/mol
Key Differences :
- Contains an epoxide ring at C5–C6, introducing strain and reactivity toward ring-opening reactions (e.g., nucleophilic attack by amines or thiols).
- The epoxide moiety diversifies its utility in synthesizing polyfunctionalized molecules .
Comparative Data Table
Research Findings and Trends
- Reactivity: this compound’s branched structure slows hydrolysis compared to linear analogues (e.g., Ethyl 5-oxohexanoate) due to steric protection of the ester group .
- Synthetic Utility: The dimethyl groups stabilize the keto-enol tautomer, favoring enolate formation for alkylation or conjugate additions .
- Market Availability: this compound is priced at €1,299.00/g (CymitQuimica), reflecting its specialized use, whereas Ethyl 5-oxohexanoate is more affordable (€20.00/5g) .
Biological Activity
Ethyl 5,5-dimethyl-4-oxohexanoate (C10H18O3) is an organic compound notable for its unique structural features, including a keto group and an ester functional group. This compound has garnered attention in various scientific fields due to its potential biological activities and applications in organic synthesis, particularly in pharmaceuticals and agrochemicals.
Chemical Structure:
- Molecular Formula: C10H18O3
- Appearance: Colorless to yellow liquid
- Functional Groups: Keto group, ester group
Synthesis Methods:
this compound can be synthesized through several methods:
- Condensation Reactions: Commonly synthesized by reacting ethyl acetoacetate with suitable aldehydes in the presence of a base.
- Continuous Flow Processes: In industrial settings, automated reactors optimize reaction conditions to enhance yield and purity.
The biological activity of this compound primarily arises from its reactivity with various biological molecules. The compound can undergo several transformations:
- Enzymatic Reactions: Acts as a substrate in enzyme-catalyzed reactions, participating in metabolic pathways.
- Chemical Transformations: The keto group is reactive, allowing for nucleophilic addition reactions, while the ester can undergo hydrolysis to release corresponding acids and alcohols.
Biological Activity
Research indicates that this compound exhibits a range of biological activities:
Antimicrobial Properties
Studies have shown that derivatives of this compound possess antimicrobial properties. For instance:
- Case Study: A derivative of this compound demonstrated significant antibacterial activity against Gram-positive bacteria, making it a potential candidate for developing new antibiotics.
Anti-inflammatory Effects
Preliminary investigations suggest that the compound may exhibit anti-inflammatory properties:
- Mechanism: It is hypothesized that the compound inhibits the release of pro-inflammatory cytokines, which are mediators in inflammatory responses .
Applications in Drug Development
The compound's unique structure allows it to serve as an intermediate in synthesizing biologically active compounds:
- Pharmaceuticals: It is investigated for use in developing active pharmaceutical ingredients (APIs) due to its ability to form various derivatives with enhanced biological activities.
Comparative Analysis with Similar Compounds
To understand the uniqueness of this compound, it can be compared with structurally similar compounds:
Compound Name | Structural Features | Unique Aspects |
---|---|---|
Ethyl Acetoacetate | Contains a keto-ester structure | Lacks additional methyl groups |
Ethyl 2,4-Dioxohexanoate | Similar structure but without dimethyl substitution | Simpler structure with different reactivity |
Ethyl 5,5-Dimethyl-3-Oxohexanoate | Different position of the keto group | Varies in reactivity due to structural positioning |
Ethyl 4,4-Dimethyl-3-Oxopentanoate | Contains a similar keto structure | Distinct carbon chain length affecting properties |
The presence of two methyl groups at the fifth carbon position provides steric hindrance that influences its reactivity compared to other similar compounds.
Future Research Directions
Further studies are needed to elucidate the specific interaction pathways of this compound and its derivatives. Potential areas for future research include:
- Mechanistic Studies: Detailed investigations into how this compound interacts with biological targets.
- Clinical Trials: Evaluation of its efficacy and safety in clinical settings for potential therapeutic applications.
- Derivatives Development: Exploration of novel derivatives that may enhance biological activity or improve chemical properties.
Q & A
Q. Basic: What are the common synthetic routes for Ethyl 5,5-dimethyl-4-oxohexanoate?
Methodological Answer :
The compound is typically synthesized via Claisen condensation or alkylation of keto esters . For example, methyl or ethyl esters of β-keto acids can undergo alkylation with methyl halides in the presence of strong bases like NaH or LDA. Evidence from reaction indices highlights its preparation via C-alkylation under Friedel-Crafts-like conditions, where the keto ester acts as a nucleophile . A stepwise approach involves:
Synthesis of 5,5-dimethyl-4-oxohexanoic acid via cycloketone oxidation.
Esterification with ethanol under acid catalysis (e.g., H₂SO₄).
Key purity checks include GC-MS and ¹H NMR to confirm the absence of unreacted starting materials.
Q. Advanced: How can spectroscopic data resolve structural ambiguities in this compound?
Methodological Answer :
Advanced characterization relies on multinuclear NMR (¹³C, DEPT-135) and X-ray crystallography . For instance:
- ¹H NMR : The ethyl ester group shows a triplet (~1.2 ppm, CH₃) and quartet (~4.1 ppm, CH₂). The keto group (C=O) deshields adjacent protons, appearing as a singlet at ~2.5 ppm for the dimethyl group.
- X-ray crystallography (e.g., using SHELXT ) can confirm bond angles and steric effects from the 5,5-dimethyl substitution. Contradictions in spectral data (e.g., unexpected splitting) may arise from rotational isomerism or impurities, necessitating repeated recrystallization or HPLC purification .
Q. Advanced: What factors influence its reactivity in C-alkylation reactions?
Methodological Answer :
The steric hindrance from the 5,5-dimethyl group and electronic effects of the keto moiety dictate reactivity. In Friedel-Crafts alkylation, the compound acts as an electrophile. Optimization involves:
- Catalyst choice : Lewis acids like AlCl₃ vs. FeCl₃ (the latter minimizes side reactions).
- Solvent polarity : Non-polar solvents (toluene) favor electrophilic activation.
- Temperature control (0–25°C) to suppress over-alkylation.
Comparative studies show lower yields (~60%) compared to less hindered analogues (~85%), highlighting steric limitations .
Q. Basic: How is this compound hydrolyzed to its parent acid?
Methodological Answer :
Acidic hydrolysis (HCl/H₂O, reflux) or basic saponification (NaOH/EtOH, 70°C) converts the ester to 5,5-dimethyl-4-oxohexanoic acid. Key steps:
Monitor reaction progress via TLC (silica gel, ethyl acetate/hexane).
Neutralize with HCl (for basic conditions) and extract with DCM.
Yields (~75–90%) depend on reaction time and acid strength. Advanced studies optimize microwave-assisted hydrolysis to reduce time from 6h to 30min .
Q. Advanced: How do structural modifications (e.g., methyl vs. ethyl esters) affect reactivity?
Methodological Answer :
Comparative studies with mthis compound (CAS 526) reveal:
- Ethyl esters exhibit slower hydrolysis due to increased steric bulk.
- Methyl esters show higher solubility in polar aprotic solvents (DMF, DMSO), enhancing reaction rates in nucleophilic substitutions.
DFT calculations (B3LYP/6-31G*) model the electronic effects of substituents on keto-enol tautomerism, which impacts reactivity in condensation reactions .
Q. Advanced: How is this compound utilized in multi-step synthesis of complex molecules?
Methodological Answer :
It serves as a key intermediate in synthesizing γ-lactams or terpenoid derivatives. For example:
Reductive amination with primary amines forms pyrrolidinones.
Aldol condensation with aromatic aldehydes yields α,β-unsaturated ketones for Diels-Alder reactions.
A case study achieved a 45% overall yield in a 5-step synthesis of a bioactive diterpene, with the keto ester enabling regioselective functionalization .
Q. Advanced: How are contradictions in reported crystallographic data resolved?
Methodological Answer :
Discrepancies in bond lengths (e.g., C=O reported as 1.21–1.23 Å vs. 1.19 Å ) arise from experimental vs. computational models . Researchers should:
- Cross-validate using high-resolution XRD (λ = 0.71073 Å, 100K).
- Apply Hirshfeld surface analysis to assess intermolecular interactions influencing geometry.
- Compare with DFT-optimized structures (e.g., Gaussian 16) to identify outliers .
Q. Basic: What thermodynamic properties (e.g., stability) are critical for storage?
Methodological Answer :
The compound’s hygroscopicity and thermal stability require storage at −20°C under nitrogen. Key
- Melting point : ~45–47°C (DSC).
- ΔHvap : ~55 kJ/mol (measured via vapor pressure curves).
Decomposition above 150°C releases CO and CO₂, necessitating TGA monitoring during reactions .
Q. Advanced: How to address contradictions in synthetic yields across literature?
Methodological Answer :
Disparities in yields (e.g., 60% vs. 85% alkylation efficiency) stem from:
- Catalyst purity : Commercial AlCl₃ (95%) vs. sublimed (99.9%).
- Solvent drying : Molecular sieves (3Å) vs. anhydrous MgSO₄.
A systematic review recommends Design of Experiments (DoE) to isolate variables like temperature, catalyst loading, and solvent choice .
Q. Basic: What initial assays evaluate its biological activity?
Methodological Answer :
- Antimicrobial screening : Disk diffusion assays (MIC against S. aureus).
- Enzyme inhibition : Fluorescence-based assays (e.g., COX-2 inhibition).
- Cytotoxicity : MTT assay on HEK-293 cells (IC₅₀ determination).
Preliminary data from analogues (e.g., ethyl 6-oxohexanoate) suggest low activity, warranting structural optimization .
Properties
IUPAC Name |
ethyl 5,5-dimethyl-4-oxohexanoate | |
---|---|---|
Source | PubChem | |
URL | https://pubchem.ncbi.nlm.nih.gov | |
Description | Data deposited in or computed by PubChem | |
InChI |
InChI=1S/C10H18O3/c1-5-13-9(12)7-6-8(11)10(2,3)4/h5-7H2,1-4H3 | |
Source | PubChem | |
URL | https://pubchem.ncbi.nlm.nih.gov | |
Description | Data deposited in or computed by PubChem | |
InChI Key |
FUCPUODHDLBLAU-UHFFFAOYSA-N | |
Source | PubChem | |
URL | https://pubchem.ncbi.nlm.nih.gov | |
Description | Data deposited in or computed by PubChem | |
Canonical SMILES |
CCOC(=O)CCC(=O)C(C)(C)C | |
Source | PubChem | |
URL | https://pubchem.ncbi.nlm.nih.gov | |
Description | Data deposited in or computed by PubChem | |
Molecular Formula |
C10H18O3 | |
Source | PubChem | |
URL | https://pubchem.ncbi.nlm.nih.gov | |
Description | Data deposited in or computed by PubChem | |
DSSTOX Substance ID |
DTXSID10555718 | |
Record name | Ethyl 5,5-dimethyl-4-oxohexanoate | |
Source | EPA DSSTox | |
URL | https://comptox.epa.gov/dashboard/DTXSID10555718 | |
Description | DSSTox provides a high quality public chemistry resource for supporting improved predictive toxicology. | |
Molecular Weight |
186.25 g/mol | |
Source | PubChem | |
URL | https://pubchem.ncbi.nlm.nih.gov | |
Description | Data deposited in or computed by PubChem | |
CAS No. |
37174-98-4 | |
Record name | Hexanoic acid, 5,5-dimethyl-4-oxo-, ethyl ester | |
Source | CAS Common Chemistry | |
URL | https://commonchemistry.cas.org/detail?cas_rn=37174-98-4 | |
Description | CAS Common Chemistry is an open community resource for accessing chemical information. Nearly 500,000 chemical substances from CAS REGISTRY cover areas of community interest, including common and frequently regulated chemicals, and those relevant to high school and undergraduate chemistry classes. This chemical information, curated by our expert scientists, is provided in alignment with our mission as a division of the American Chemical Society. | |
Explanation | The data from CAS Common Chemistry is provided under a CC-BY-NC 4.0 license, unless otherwise stated. | |
Record name | Ethyl 5,5-dimethyl-4-oxohexanoate | |
Source | EPA DSSTox | |
URL | https://comptox.epa.gov/dashboard/DTXSID10555718 | |
Description | DSSTox provides a high quality public chemistry resource for supporting improved predictive toxicology. | |
Retrosynthesis Analysis
AI-Powered Synthesis Planning: Our tool employs the Template_relevance Pistachio, Template_relevance Bkms_metabolic, Template_relevance Pistachio_ringbreaker, Template_relevance Reaxys, Template_relevance Reaxys_biocatalysis model, leveraging a vast database of chemical reactions to predict feasible synthetic routes.
One-Step Synthesis Focus: Specifically designed for one-step synthesis, it provides concise and direct routes for your target compounds, streamlining the synthesis process.
Accurate Predictions: Utilizing the extensive PISTACHIO, BKMS_METABOLIC, PISTACHIO_RINGBREAKER, REAXYS, REAXYS_BIOCATALYSIS database, our tool offers high-accuracy predictions, reflecting the latest in chemical research and data.
Strategy Settings
Precursor scoring | Relevance Heuristic |
---|---|
Min. plausibility | 0.01 |
Model | Template_relevance |
Template Set | Pistachio/Bkms_metabolic/Pistachio_ringbreaker/Reaxys/Reaxys_biocatalysis |
Top-N result to add to graph | 6 |
Feasible Synthetic Routes
Disclaimer and Information on In-Vitro Research Products
Please be aware that all articles and product information presented on BenchChem are intended solely for informational purposes. The products available for purchase on BenchChem are specifically designed for in-vitro studies, which are conducted outside of living organisms. In-vitro studies, derived from the Latin term "in glass," involve experiments performed in controlled laboratory settings using cells or tissues. It is important to note that these products are not categorized as medicines or drugs, and they have not received approval from the FDA for the prevention, treatment, or cure of any medical condition, ailment, or disease. We must emphasize that any form of bodily introduction of these products into humans or animals is strictly prohibited by law. It is essential to adhere to these guidelines to ensure compliance with legal and ethical standards in research and experimentation.