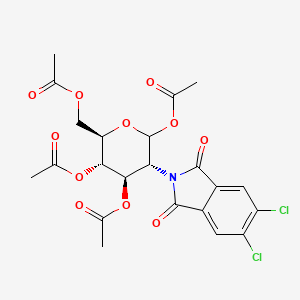
1,3,4,6-Tetra-O-acetyl-2-deoxy-2-(4,5-dichlorophthalimido)-D-glucopyranose
- Click on QUICK INQUIRY to receive a quote from our team of experts.
- With the quality product at a COMPETITIVE price, you can focus more on your research.
Description
1,3,4,6-Tetra-O-acetyl-2-deoxy-2-(4,5-dichlorophthalimido)-D-glucopyranose is a synthetic compound with the chemical formula C₂₂H₂₁Cl₂NO₁₁ . It is a derivative of glucose, specifically an acetylated form, and contains phthalimide substituents. The compound is used in various research contexts due to its unique structure and potential applications .
Scientific Research Applications
Synthesis and Biological Activity
The compound 1,3,4,6-tetra-O-acetyl-2-deoxy-2-(4,5-dichlorophthalimido)-D-glucopyranose is a synthetic intermediate that has been utilized in the synthesis of various glycosylated compounds with potential biological activities. For instance, derivatives of this compound have been synthesized for the induction of apoptosis in B cell chronic leukemia, highlighting its relevance in the development of antitumor agents. Glycosylation reactions involving this compound, under mild conditions using silver triflate as a promoter, have yielded diosgenyl glycosides showing promising antitumor activities, especially in increasing apoptotic B cells in chronic lymphotic leukemia (B-CLL) patients (Myszka et al., 2003).
Chemical Synthesis Techniques
The chemical synthesis and modification of this compound have been explored extensively. Techniques such as acetylation, N-phthaloylation, and the use of Lewis acids for anomeric chlorination have been employed to synthesize various derivatives. These processes are critical for producing pure β-glucosyl chloride derivatives, which are crucial intermediates for further chemical transformations (Cao et al., 2013). Additionally, the structural characterization and optimization of reaction conditions for synthesizing N-nitromaticacyl derivatives of this compound have been investigated, providing insights into its chemical behavior and potential applications in synthesizing novel glycosylated compounds (Wei–wei, 2013).
Methodological Developments
Recent methodological advancements have enabled the one-pot synthesis of this compound from glucosamine glycosyl donors, showcasing the efficiency of anomeric O-acylation processes. This approach not only simplifies the synthesis of such complex molecules but also opens avenues for the creation of a wide range of glucosamine derivatives, expanding its applicability in medicinal chemistry and drug development (Pertel et al., 2018).
Glycosylation and Drug Development
The role of this compound extends to glycosylation reactions, serving as a glycosyl donor for the synthesis of diosgenyl 2-amino-2-deoxy-β-D-glucopyranoside hydrochloride, a compound with potential antitumor properties. Such reactions not only demonstrate the compound's utility in synthesizing biologically active molecules but also highlight its significance in the context of drug development and synthetic biology (Bednarczyk et al., 2000).
properties
IUPAC Name |
[(2R,3S,4R,5R)-3,4,6-triacetyloxy-5-(5,6-dichloro-1,3-dioxoisoindol-2-yl)oxan-2-yl]methyl acetate |
Source
|
---|---|---|
Source | PubChem | |
URL | https://pubchem.ncbi.nlm.nih.gov | |
Description | Data deposited in or computed by PubChem | |
InChI |
InChI=1S/C22H21Cl2NO11/c1-8(26)32-7-16-18(33-9(2)27)19(34-10(3)28)17(22(36-16)35-11(4)29)25-20(30)12-5-14(23)15(24)6-13(12)21(25)31/h5-6,16-19,22H,7H2,1-4H3/t16-,17-,18-,19-,22?/m1/s1 |
Source
|
Source | PubChem | |
URL | https://pubchem.ncbi.nlm.nih.gov | |
Description | Data deposited in or computed by PubChem | |
InChI Key |
LHCNZORUZHETLY-SWHXLWHISA-N |
Source
|
Source | PubChem | |
URL | https://pubchem.ncbi.nlm.nih.gov | |
Description | Data deposited in or computed by PubChem | |
Canonical SMILES |
CC(=O)OCC1C(C(C(C(O1)OC(=O)C)N2C(=O)C3=CC(=C(C=C3C2=O)Cl)Cl)OC(=O)C)OC(=O)C |
Source
|
Source | PubChem | |
URL | https://pubchem.ncbi.nlm.nih.gov | |
Description | Data deposited in or computed by PubChem | |
Isomeric SMILES |
CC(=O)OC[C@@H]1[C@H]([C@@H]([C@H](C(O1)OC(=O)C)N2C(=O)C3=CC(=C(C=C3C2=O)Cl)Cl)OC(=O)C)OC(=O)C |
Source
|
Source | PubChem | |
URL | https://pubchem.ncbi.nlm.nih.gov | |
Description | Data deposited in or computed by PubChem | |
Molecular Formula |
C22H21Cl2NO11 |
Source
|
Source | PubChem | |
URL | https://pubchem.ncbi.nlm.nih.gov | |
Description | Data deposited in or computed by PubChem | |
DSSTOX Substance ID |
DTXSID30584101 |
Source
|
Record name | 1,3,4,6-Tetra-O-acetyl-2-deoxy-2-(5,6-dichloro-1,3-dioxo-1,3-dihydro-2H-isoindol-2-yl)-D-glucopyranose | |
Source | EPA DSSTox | |
URL | https://comptox.epa.gov/dashboard/DTXSID30584101 | |
Description | DSSTox provides a high quality public chemistry resource for supporting improved predictive toxicology. | |
Molecular Weight |
546.3 g/mol |
Source
|
Source | PubChem | |
URL | https://pubchem.ncbi.nlm.nih.gov | |
Description | Data deposited in or computed by PubChem | |
Product Name |
1,3,4,6-Tetra-O-acetyl-2-deoxy-2-(4,5-dichlorophthalimido)-D-glucopyranose | |
CAS RN |
308796-43-2 |
Source
|
Record name | 1,3,4,6-Tetra-O-acetyl-2-deoxy-2-(5,6-dichloro-1,3-dioxo-1,3-dihydro-2H-isoindol-2-yl)-D-glucopyranose | |
Source | EPA DSSTox | |
URL | https://comptox.epa.gov/dashboard/DTXSID30584101 | |
Description | DSSTox provides a high quality public chemistry resource for supporting improved predictive toxicology. | |
Disclaimer and Information on In-Vitro Research Products
Please be aware that all articles and product information presented on BenchChem are intended solely for informational purposes. The products available for purchase on BenchChem are specifically designed for in-vitro studies, which are conducted outside of living organisms. In-vitro studies, derived from the Latin term "in glass," involve experiments performed in controlled laboratory settings using cells or tissues. It is important to note that these products are not categorized as medicines or drugs, and they have not received approval from the FDA for the prevention, treatment, or cure of any medical condition, ailment, or disease. We must emphasize that any form of bodily introduction of these products into humans or animals is strictly prohibited by law. It is essential to adhere to these guidelines to ensure compliance with legal and ethical standards in research and experimentation.