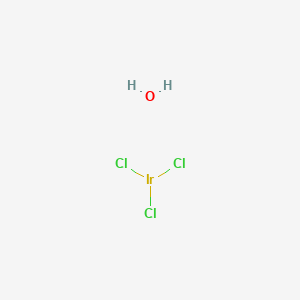
Iridium(III) chloride hydrate
Overview
Description
Iridium(III) chloride hydrate: is an inorganic compound with the chemical formula IrCl₃·xH₂O. It is a dark green to black hygroscopic solid that is widely used as a precursor in various chemical reactions and industrial applications . This compound is a common starting material for iridium chemistry and is known for its stability and reactivity.
Mechanism of Action
Target of Action
Iridium(III) chloride hydrate is primarily used as a catalyst in organic synthesis . It is also used as a precursor in the preparation of other iridium compounds .
Mode of Action
As a catalyst, this compound facilitates chemical reactions without being consumed in the process. It can accelerate reactions by providing an alternative reaction pathway with a lower activation energy . For instance, it can be used to synthesize β-amino alcohols by aminolysis of epoxides .
Biochemical Pathways
The specific biochemical pathways affected by this compound depend on the reactions it catalyzes. For example, in the aminolysis of epoxides, it could affect the pathways involving β-amino alcohols .
Pharmacokinetics
Instead, it facilitates reactions in the environment where it is applied .
Result of Action
The result of this compound’s action is the acceleration of certain chemical reactions. For example, it can increase the rate of aminolysis of epoxides, leading to the production of β-amino alcohols .
Action Environment
The efficacy and stability of this compound can be influenced by various environmental factors. For instance, it is soluble in water and alcohol, which can affect its distribution and reactivity . It is also hygroscopic, meaning it absorbs moisture from the air, which can impact its physical state and reactivity . Furthermore, it decomposes at high temperatures (763°C), which can limit its use in certain conditions .
Biochemical Analysis
Biochemical Properties
Iridium(III) chloride hydrate plays a significant role in biochemical reactions, particularly as a catalyst. It interacts with various enzymes, proteins, and other biomolecules to facilitate oxidation reactions. For instance, it is used in the preparation of iridium nanocatalysts, which are employed in hydrodeoxygenation reactions . The compound’s interaction with biomolecules often involves the formation of coordination complexes, where the iridium ion binds to specific sites on the enzyme or protein, altering its activity and facilitating the desired reaction.
Cellular Effects
This compound has been shown to influence various cellular processes. It can affect cell signaling pathways, gene expression, and cellular metabolism. For example, studies have demonstrated that this compound can induce changes in the expression of certain genes involved in oxidative stress responses . Additionally, the compound’s catalytic properties can impact cellular metabolism by altering the rates of specific biochemical reactions.
Molecular Mechanism
The molecular mechanism of action of this compound involves its ability to form coordination complexes with biomolecules. These interactions can lead to enzyme inhibition or activation, depending on the specific enzyme and the nature of the binding interaction. For instance, this compound can inhibit certain enzymes by binding to their active sites, preventing substrate access and thus reducing enzyme activity . Conversely, it can also activate other enzymes by inducing conformational changes that enhance their catalytic efficiency.
Temporal Effects in Laboratory Settings
In laboratory settings, the effects of this compound can change over time due to its stability and degradation properties. The compound is generally stable under standard laboratory conditions, but prolonged exposure to light and air can lead to its gradual degradation . Long-term studies have shown that this compound can have sustained effects on cellular function, particularly in in vitro settings where it is used as a catalyst in biochemical assays.
Dosage Effects in Animal Models
The effects of this compound vary with different dosages in animal models. At low doses, the compound can enhance certain biochemical reactions without causing significant toxicity. At higher doses, this compound can induce toxic effects, including oxidative stress and damage to vital organs . Studies in Wistar rats have shown that high doses of this compound can lead to significant accumulation of the metal in the kidneys and liver, resulting in adverse health effects .
Metabolic Pathways
This compound is involved in various metabolic pathways, particularly those related to oxidative stress and detoxification. The compound interacts with enzymes such as catalase and superoxide dismutase, which play crucial roles in mitigating oxidative damage . Additionally, this compound can influence metabolic flux by altering the activity of key enzymes involved in energy production and redox balance.
Transport and Distribution
Within cells and tissues, this compound is transported and distributed through interactions with specific transporters and binding proteins. The compound can be taken up by cells via endocytosis and subsequently distributed to various cellular compartments . Its localization within cells is influenced by its ability to form coordination complexes with intracellular proteins, which can affect its accumulation and activity.
Subcellular Localization
This compound exhibits specific subcellular localization patterns, often accumulating in organelles such as mitochondria and lysosomes. This localization is mediated by targeting signals and post-translational modifications that direct the compound to these compartments . The activity and function of this compound can be influenced by its subcellular localization, as the microenvironment within different organelles can affect its catalytic properties and interactions with biomolecules.
Preparation Methods
Synthetic Routes and Reaction Conditions:
From Iridium Metal: Iridium metal is reacted with chlorine gas at high temperatures (around 650°C) to produce iridium(III) chloride. The reaction is as follows: [ \text{Ir} + 3\text{Cl}_2 \rightarrow \text{IrCl}_3 ]
From Hydrated Iridium(III) Oxide: Hydrated iridium(III) oxide is heated with hydrochloric acid to produce iridium(III) chloride hydrate.
Industrial Production Methods:
Mechanochemical Synthesis: A two-step mechanochemical protocol using ball milling enables the rapid and efficient synthesis of various tris-cyclometalated iridium(III) complexes from this compound without the use of significant amounts of organic solvent.
Chlorination Process: Iridium metal powder is placed in a porcelain boat and heated in the presence of chlorine gas to produce anhydrous iridium(III) chloride.
Chemical Reactions Analysis
Types of Reactions:
Oxidation: Iridium(III) chloride can be oxidized to form hexachloroiridate(IV) in the presence of aqua regia.
Reduction: It can be reduced to iridium metal using hydrogen gas.
Substitution: It reacts with ammonia to form ammine complexes, such as pentaamminechloroiridium(III) chloride.
Common Reagents and Conditions:
Ammonia: Reacts with iridium(III) chloride hydrate to form ammine complexes.
Hydrochloric Acid: Used in the preparation of this compound from hydrated iridium(III) oxide.
Major Products Formed:
Hexachloroiridate(IV): Formed in the presence of aqua regia.
Ammine Complexes: Such as pentaamminechloroiridium(III) chloride.
Scientific Research Applications
Chemistry:
Synthesis of Iridium Nanoparticles: It serves as a precursor for the synthesis of iridium nanoparticles, which are used in various catalytic applications.
Biology and Medicine:
Bioimaging Probes: Tris-cyclometalated iridium(III) complexes derived from this compound are used as bioimaging probes.
Photoredox Catalysts: These complexes are also employed as photoredox catalysts in biological systems.
Industry:
Electroplating: this compound is used in iridium electroplating to produce corrosion-resistant coatings.
Manufacture of Electrodes: It is used in the manufacture of electrodes for various industrial applications.
Comparison with Similar Compounds
Rhodium(III) Chloride: Similar in structure and reactivity, but rhodium compounds are generally less stable and less reactive than iridium compounds.
Platinum(II) Chloride: Shares similar catalytic properties but differs in its oxidation states and reactivity patterns.
Uniqueness:
Properties
IUPAC Name |
trichloroiridium;hydrate | |
---|---|---|
Source | PubChem | |
URL | https://pubchem.ncbi.nlm.nih.gov | |
Description | Data deposited in or computed by PubChem | |
InChI |
InChI=1S/3ClH.Ir.H2O/h3*1H;;1H2/q;;;+3;/p-3 | |
Source | PubChem | |
URL | https://pubchem.ncbi.nlm.nih.gov | |
Description | Data deposited in or computed by PubChem | |
InChI Key |
MJRFDVWKTFJAPF-UHFFFAOYSA-K | |
Source | PubChem | |
URL | https://pubchem.ncbi.nlm.nih.gov | |
Description | Data deposited in or computed by PubChem | |
Canonical SMILES |
O.Cl[Ir](Cl)Cl | |
Source | PubChem | |
URL | https://pubchem.ncbi.nlm.nih.gov | |
Description | Data deposited in or computed by PubChem | |
Molecular Formula |
Cl3H2IrO | |
Source | PubChem | |
URL | https://pubchem.ncbi.nlm.nih.gov | |
Description | Data deposited in or computed by PubChem | |
Molecular Weight |
316.59 g/mol | |
Source | PubChem | |
URL | https://pubchem.ncbi.nlm.nih.gov | |
Description | Data deposited in or computed by PubChem | |
CAS No. |
14996-61-3 | |
Record name | Iridium trichloride hydrate | |
Source | CAS Common Chemistry | |
URL | https://commonchemistry.cas.org/detail?cas_rn=14996-61-3 | |
Description | CAS Common Chemistry is an open community resource for accessing chemical information. Nearly 500,000 chemical substances from CAS REGISTRY cover areas of community interest, including common and frequently regulated chemicals, and those relevant to high school and undergraduate chemistry classes. This chemical information, curated by our expert scientists, is provided in alignment with our mission as a division of the American Chemical Society. | |
Explanation | The data from CAS Common Chemistry is provided under a CC-BY-NC 4.0 license, unless otherwise stated. | |
Record name | Iridium(III) chloride hydrate | |
Source | European Chemicals Agency (ECHA) | |
URL | https://echa.europa.eu/information-on-chemicals | |
Description | The European Chemicals Agency (ECHA) is an agency of the European Union which is the driving force among regulatory authorities in implementing the EU's groundbreaking chemicals legislation for the benefit of human health and the environment as well as for innovation and competitiveness. | |
Explanation | Use of the information, documents and data from the ECHA website is subject to the terms and conditions of this Legal Notice, and subject to other binding limitations provided for under applicable law, the information, documents and data made available on the ECHA website may be reproduced, distributed and/or used, totally or in part, for non-commercial purposes provided that ECHA is acknowledged as the source: "Source: European Chemicals Agency, http://echa.europa.eu/". Such acknowledgement must be included in each copy of the material. ECHA permits and encourages organisations and individuals to create links to the ECHA website under the following cumulative conditions: Links can only be made to webpages that provide a link to the Legal Notice page. | |
Q1: What are the typical synthetic applications of iridium(III) chloride hydrate?
A: this compound serves as a common starting material for synthesizing a wide range of tris-cyclometalated iridium(III) complexes. These complexes have garnered significant interest due to their potential applications in diverse areas, including organic light-emitting diodes (OLEDs) [], photoredox catalysts, and bioimaging probes [].
Q2: What are the limitations of traditional methods for synthesizing these iridium(III) complexes?
A: Traditional synthetic approaches often involve lengthy reaction times, require substantial quantities of high-boiling solvents, involve multiple synthetic steps, and necessitate the use of inert-gas-line techniques []. These requirements pose drawbacks in terms of production costs and environmental impact.
Q3: How does mechanochemistry offer a more efficient alternative for synthesizing these complexes?
A: Research demonstrates that a two-step mechanochemical protocol employing ball milling allows for the rapid and efficient synthesis of various tris-cyclometalated iridium(III) complexes starting from the readily available this compound []. This solid-state method eliminates the need for large volumes of organic solvents and can be performed in an air atmosphere, making it a more sustainable and cost-effective approach.
Q4: Can you provide an example of a specific iridium complex synthesized using this compound and its potential application?
A: A specific example is the synthesis of [Iridium, Methylbis(2,4‐pentanedionato‐κO, κO′) (Pyridine)-] []. This complex, synthesized through a multi-step process starting from this compound, acts as a catalyst for C-H activation reactions involving alkyl, benzyl, and aryl substrates [].
Q5: Are there any structural studies available on complexes derived from this compound?
A: Yes, several studies delve into the structural characterization of iridium complexes derived from this compound. For instance, one study reports the crystal structures of mer-Trichloro(1,2-ethanediamine)(2-aminoethylammonium)this compound, mer-[Ir(en)(enH)Cl3]Cl.H2O, and its conjugate base, mer-[Ir(en)(en*)Cl3] [].
Q6: Beyond individual complexes, are there examples of unique architectures accessible using this compound as a starting point?
A: Research highlights the synthesis of inherently dinuclear iridium(III) meso architectures []. These unique structures are obtained through the cyclometalation of calix[4]arene bis(aryltriazoles) with this compound. The resulting complexes feature an Ir2Cl2 core bridged by two (C^N)2 ligands, with the chloride bridges amenable to replacement by ancillary ligands [].
Q7: Can you elaborate on the impact of ligand modification on the properties of iridium(III) complexes?
A: Research on phosphorescent systems based on iridium(III) complexes emphasizes the significant influence of ligand structures on the complexes' electro-optical properties []. The choice of cyclometallating and ancillary ligands allows for fine-tuning of emission color, luminescence efficiency, and lifetime []. For example, modifications to the periphery of S-terpyridine ligands have been shown to induce subtle changes in optical properties, while alterations to the central substituent or the terpyridine ring conjunction lead to more pronounced effects on emission behavior [].
Q8: Are there examples of functionalization strategies for these iridium complexes, and what are their potential advantages?
A: The use of "click" chemistry with acetylene and azide functionalities allows for the synthesis of novel cyclometallating ligands, which can be further incorporated into iridium complexes []. This approach enables the introduction of specific functionalities and facilitates the tuning of electro-optical properties []. Additionally, employing acetoacetate derivatives as ancillary ligands offers a versatile platform for introducing diverse functional groups through straightforward transesterification reactions []. These functional groups, such as oxetane and methacrylate, allow for incorporating the phosphorescent iridium(III) complexes into polymeric assemblies, expanding their potential applications in materials science [].
Q9: Are there studies investigating the stability of iridium(III) complexes under specific conditions?
A: Research on incorporating oxetane-equipped iridium(III) phosphors into oxetane-based crosslinkable matrices highlights the importance of complex stability, particularly under acidic conditions, for device performance []. While this specific example focuses on material stability within a device context, understanding and potentially enhancing the stability of iridium complexes remain crucial for their broader application in various fields.
Q10: Is there research exploring the in vivo behavior of iridium compounds?
A: One study investigates the sub-chronic oral exposure to this compound in female Wistar rats []. This research focuses on understanding the distribution and excretion of iridium in a living organism, providing insights into the compound's pharmacokinetics. The findings indicate that iridium primarily accumulates in the kidneys and spleen, with excretion predominantly occurring through feces. Notably, higher doses led to increased iridium levels in serum and urine, suggesting a potential shift in elimination pathways at elevated exposures [].
Disclaimer and Information on In-Vitro Research Products
Please be aware that all articles and product information presented on BenchChem are intended solely for informational purposes. The products available for purchase on BenchChem are specifically designed for in-vitro studies, which are conducted outside of living organisms. In-vitro studies, derived from the Latin term "in glass," involve experiments performed in controlled laboratory settings using cells or tissues. It is important to note that these products are not categorized as medicines or drugs, and they have not received approval from the FDA for the prevention, treatment, or cure of any medical condition, ailment, or disease. We must emphasize that any form of bodily introduction of these products into humans or animals is strictly prohibited by law. It is essential to adhere to these guidelines to ensure compliance with legal and ethical standards in research and experimentation.