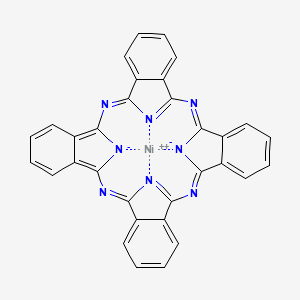
Nickel phthalocyanine
- Click on QUICK INQUIRY to receive a quote from our team of experts.
- With the quality product at a COMPETITIVE price, you can focus more on your research.
Overview
Description
Nickel phthalocyanine (NiPc) is a macrocyclic complex with a nickel ion coordinated to a phthalocyanine ligand, forming a planar structure. Its chemical formula is Ni(C₃₂H₁₆N₈), and it exhibits unique electronic, optical, and magnetic properties due to the conjugation of the phthalocyanine ring and the redox-active nickel center . NiPc is synthesized via cyclotetramerization of phthalonitrile derivatives in the presence of nickel salts, often under inert conditions . Key applications include catalysis, gas sensing, organic electronics, and energy storage, driven by its tunable bandgap (~1.5–2.0 eV) and high thermal stability .
Preparation Methods
Nickel phthalocyanine can be synthesized through several methods, including:
Tetramerization of Phthalonitrile: This method involves the tetramerization of phthalonitrile in the presence of a nickel salt, such as nickel acetate, under high-temperature conditions.
Solvothermal Synthesis: This method involves the reaction of phthalonitrile with a nickel salt in a solvent under solvothermal conditions.
Sublimation: this compound can also be purified by sublimation under high vacuum in a slow stream of carbon dioxide.
Chemical Reactions Analysis
Complexation
Nickel phthalocyanine demonstrates the ability to coordinate with various metal cations (such as Fe and Co) through its pyrrolic nitrogen atoms. These complexes exhibit stable metal-ligand interactions, enabling applications across diverse fields .
Reactions with Chemical Vapors
This compound interacts with various chemical vapors, leading to changes in its electrical and spectroscopic properties :
-
Reducing Vapors: Exposure to reducing vapors like diethylamine can cause anomalous behavior in this compound due to alterations in its spectroscopic transitions and magnetic states .
-
Oxidizing Vapors: Exposure to oxidizing vapors like bromine leads to significant changes in the vibrational bands of this compound .
The variations in electronic transition levels after vapor exposure, studied using UV-Visible spectroscopy, confirm electrical sensing results, indicating the central metal atom's significant role in the interaction mechanism .
Electrocatalytic Reactions
This compound derivatives are effective electrocatalysts for various reactions . Studies have identified this compound derivatives as effective pH-universal electrocatalysts for hydrogen peroxide production after being heterogenized on nitrogen-decorated carbon . Increased conjugation degrees lead to boosted selectivity, which is explained by the regulated d-band center, optimizing the binding energy of the reaction intermediate, reducing the energy barrier for oxygen reduction, and leading to optimized hydrogen peroxide selectivity .
A graphite-conjugated this compound demonstrates electrocatalytic performance in both H-type and flow cells .
Photocatalytic Reactions
Nickel(II) phthalocyanine (NiPc) can be used in photocatalytic systems for hydrogen production . When combined with graphene oxide (GO) and TiO2, NiPc enhances the photocatalytic reaction rate as a sensitizing agent . The reaction releases hydrogen at a specific rate, and the activation energy is calculated to be relatively low for the reaction with NiPc@GO/TiO2 .
Kinetic Studies
Kinetic studies of this compound synthesis reveal that the activation energies are low, indicating a transport mechanism is more prevalent than an entirely reactive mechanism . The cyclization reaction to form the metallic phthalocyanine from four phthalonitrile molecules with a metal is slow .
Table 1: Kinetic Parameters for Ni/Al2O3 in Batch and Parallel-Plate Reactors
Parameter | Batch Reactor | Parallel-Plate Reactor |
---|---|---|
Activation Energy (Ea) (J/mol) | 24,615 | 21,475 |
Pre-exponential factor (k0) | 0.1658 | 3.187 x 10-2 |
Scientific Research Applications
Nickel Phthalocyanine (Ni-Pc) is a macrocyclic compound with a wide array of applications stemming from its unique chemical and physical properties . Its chemical stability, high electrocatalytic activity, and supramolecular arrangement make it suitable for use in thin films, solar cells, gas detectors, and electrochemical ammonia synthesis .
Scientific Research Applications
- Electrocatalysis this compound derivatives have been identified as effective pH-universal electrocatalysts for hydrogen peroxide production when heterogenized on nitrogen-decorated carbon . Increased conjugation degrees lead to boosted selectivity, which can be explained by the regulated d-band center, which optimizes the binding energy of the reaction intermediate, reducing the energy barrier for oxygen reduction and leading to optimized H2O2 selectivity .
- Electrochemical Ammonia Synthesis this compound nanorods exhibit an NH3 yield rate of about 85 μg h−1 mgcat−1 and a faradaic efficiency (FE) of 25% at −0.3 V vs. RHE, with long-term stability up to 30 hours .
- Solar Cells this compound is tested to be applied in solar cells as a transfer asset mediator . It can also be used in perovskite solar cells .
- Gas detection Due to its excellent thermal-chemical stabilities as a semiconductor property, this compound is used in the development of gas detector devices .
- Antibacterial Activity Different concentrations of Nickel (II) Phthalocyanine have been studied regarding the effect of antibacterial activity against two species of bacteria Escherichia coli and Staphylococcus aureus, using disk-diffusion methods in Mueller–Hinton agar .
Data Tables and Case Studies
Table 1: Electrocatalytic Activity of this compound Derivatives
Case Study: this compound Thin Films for Solar Cells
This compound thin films have been investigated for their potential in solar cell applications . The study of Ni-Phthalocyanine thin films reveals that the increase in substrate temperature causes a decrease in absorbance, except in the range of 350-400 K, where an inverse behavior for Q band and B band is observed . The lowest value for Eg is at Ts 400 K, which is the same result found by Tauc equation . P-type films have been observed by Hall effects, whereas the carriers mobility (µ) goes up from 0.03 (cm2/v.s.) to 99.42 (cm2/v.s.) by increasing Ts from (325 - 450) K for prepared films except at 300 K .
Case Study: Water-Vapour-Enhanced Oxidation
Mechanism of Action
The mechanism of action of nickel phthalocyanine involves its ability to act as a catalyst in various chemical reactions. The central nickel ion plays a crucial role in facilitating electron transfer processes. For example, in the electrochemical reduction of carbon dioxide, the nickel ion enhances the electron density at the active site, reducing the adsorption energy barrier of intermediates and improving catalytic performance . Additionally, the conjugated structure of this compound allows for efficient charge transport, making it suitable for applications in optoelectronic devices .
Comparison with Similar Compounds
Electronic and Charge Transport Properties
NiPc demonstrates superior charge carrier mobility compared to other metal phthalocyanines (MPcs). For instance, NiPc-based covalent organic frameworks (COFs) exhibit a carrier mobility of 8.1 cm² V⁻¹ s⁻¹, significantly higher than CuPc (3.2 cm² V⁻¹ s⁻¹) and ZnPc (1.5 cm² V⁻¹ s⁻¹) (Table 1) . This is attributed to the alignment of nickel’s d-orbitals with the π-system of the phthalocyanine ring, facilitating efficient electron delocalization.
Table 1: Electronic Properties of Metal Phthalocyanines
Compound | Bandgap (eV) | Carrier Mobility (cm² V⁻¹ s⁻¹) | Reference |
---|---|---|---|
NiPc | 1.6 | 8.1 | |
CuPc | 1.8 | 3.2 | |
ZnPc | 2.1 | 1.5 |
Table 2: Sensing Performance of MPc Films
Compound | Analyte | Sensitivity (ΔI/I₀) | Detection Limit | Reference |
---|---|---|---|---|
NiPc | Diethylamine | 1.8 | 5 ppm | |
CuPc | Benzene | 0.9 | 10 ppm | |
CoPc | Humidity | 0.75 (ΔC/C₀) | 5% RH |
Catalytic Activity
NiPc excels in electrocatalytic CO₂ reduction, achieving a Faradaic efficiency of 92% for CO production at −0.7 V vs. RHE, surpassing FePc (75%) and CuPc (68%) (Table 3) . This is linked to the optimal binding energy of *COOH intermediates on the Ni center .
Table 3: Electrocatalytic CO₂ Reduction Performance
Compound | Product | Faradaic Efficiency (%) | Overpotential (mV) | Reference |
---|---|---|---|---|
NiPc | CO | 92 | 350 | |
FePc | CO | 75 | 420 | |
CuPc | CH₄ | 68 | 500 |
Magnetic Properties
NiPc uniquely forms magnetic nanocomposites when doped with potassium, yielding metallic nickel nanoparticles (~40 nm) embedded in a phthalocyanine matrix. These composites exhibit a saturation magnetization of 45 emu/g and coercivity of 200 Oe, whereas undoped NiPc is diamagnetic .
Table 4: Performance of MPc-Based Solar Cells
Compound | Device Type | Efficiency (%) | Stability (h) | Reference |
---|---|---|---|---|
NiPc | Perovskite Solar Cell | 21.23 | 500 | |
CuPc | Organic Solar Cell | 18.5 | 300 | |
MgPc | Dye-Sensitized Cell | 16.8 | 200 |
Biological Activity
Nickel phthalocyanine (NiPc) is a metal complex with significant biological activity, making it a subject of interest in various fields such as medicine, environmental science, and materials chemistry. This article explores the biological properties of NiPc, including its anticancer potential, antibacterial effects, and photocatalytic applications.
This compound is a synthetic compound belonging to the phthalocyanine family, characterized by a large planar structure that facilitates interactions with biological molecules. Its unique electronic properties are attributed to the central nickel ion and the conjugated macrocyclic system, which enable it to participate in redox reactions and act as a photosensitizer.
2. Anticancer Activity
Recent studies have highlighted the potential of NiPc as an anticancer agent. Research indicates that NiPc can intercalate with DNA, disrupting its structure and function, which is crucial for cancer cell proliferation.
Case Study: Interaction with DNA
A study conducted by Arslantas et al. demonstrated that a synthesized Ni(II) phthalocyanine complex exhibited strong binding affinity to calf thymus DNA. The binding mechanism was primarily intercalative, confirmed through various techniques:
- Absorption Spectroscopy : Changes in UV-Vis spectra indicated strong interactions between NiPc and DNA.
- Fluorescence Titration : Increased fluorescence intensity was observed with rising concentrations of DNA.
- Viscosity Measurements : The viscosity of DNA increased upon addition of NiPc, suggesting intercalation.
The binding constant Kb was determined through titration experiments, further supporting the intercalative mechanism .
3. Antibacterial Properties
NiPc has also been evaluated for its antibacterial activity against various pathogens. A notable study assessed its efficacy against Escherichia coli and Staphylococcus aureus using the disk diffusion method.
Table 1: Antibacterial Activity of this compound
Concentration (µg/mL) | E. coli Inhibition Zone (mm) | S. aureus Inhibition Zone (mm) |
---|---|---|
10 | 12 | 10 |
25 | 18 | 15 |
50 | 25 | 20 |
The results indicated that higher concentrations of NiPc resulted in larger inhibition zones for both bacterial strains, demonstrating its potential as an antibacterial agent .
4. Photocatalytic Applications
In addition to its biological applications, NiPc has been integrated into photocatalytic systems for environmental remediation. A study reported the use of NiPc in combination with graphene oxide and titanium dioxide (TiO2) for the degradation of formic acid under visible light.
Key Findings:
- The photocatalytic system achieved a hydrogen production rate of 1.38 mmol h−1.
- The presence of NiPc enhanced the reaction rate by acting as a sensitizing agent.
- Stability tests showed no significant leaching of Ni from the catalyst during reactions, indicating its potential for repeated use in catalytic applications .
5. Conclusion
This compound exhibits promising biological activities, particularly in anticancer and antibacterial applications. Its ability to interact with DNA and inhibit bacterial growth positions it as a valuable compound in medicinal chemistry. Moreover, its photocatalytic properties open avenues for environmental applications. Future research should focus on optimizing its formulations and exploring its mechanisms further to enhance efficacy and broaden its applications.
Q & A
Basic Research Questions
Q. What experimental methodologies are recommended for synthesizing nickel phthalocyanine and confirming its structural integrity?
To synthesize this compound, common approaches include solvothermal reactions (e.g., boronate esterification for covalent organic frameworks) and pulsed laser deposition for thin films . Structural confirmation requires a combination of spectroscopic techniques:
- FT-IR and NMR to verify functional groups and substituent positions .
- X-ray absorption spectroscopy (XAS) to analyze the coordination environment of the nickel center .
- UV-Vis spectroscopy to assess electronic transitions and π-π stacking behavior .
For reproducibility, document solvent systems, temperature, and catalyst ratios in detail, and cross-validate results with elemental analysis .
Q. How can researchers fabricate this compound thin films, and what parameters influence their electrical properties?
Thin films are typically fabricated via pulsed laser deposition or spin-coating. Key parameters include:
- Substrate temperature and deposition rate , which affect crystallinity and surface morphology .
- Film thickness (e.g., 50–200 nm), which influences electrical conductivity due to variations in grain boundaries and charge carrier mobility .
Characterize films using atomic force microscopy (AFM) for surface roughness and Hall effect measurements for conductivity .
Q. What are the best practices for ensuring reproducibility in this compound synthesis?
- Provide detailed protocols for solvent selection, reaction time, and purification steps (e.g., Soxhlet extraction for covalent organic frameworks) .
- Include supplementary data (e.g., FT-IR peaks, NMR shifts) in publications to enable cross-validation .
- Use standardized characterization workflows, such as thermogravimetric analysis (TGA) for thermal stability and cyclic voltammetry for redox behavior .
Advanced Research Questions
Q. How can substituents and conjugation strategies optimize this compound for electrocatalytic CO₂ reduction?
- Substituent effects : Electron-withdrawing groups (e.g., nitro or carboxyl) enhance the nickel center’s electrophilicity, improving CO₂ adsorption and activation .
- Conjugation strategies : Graphite conjugation (e.g., Ni(NH₂)₈Pc-GC) enhances charge transfer efficiency, achieving high current densities (>200 mA/cm²) in flow cells .
- Experimental design : Use in situ XAS to monitor structural changes during catalysis and correlate with Faradaic efficiency metrics .
Q. How should researchers resolve contradictions in reported catalytic activities of this compound across studies?
Contradictions often arise from variations in:
- Synthetic conditions (e.g., solvent polarity affecting aggregation states) .
- Electrochemical setups (e.g., H-cell vs. flow cell configurations altering mass transport) .
- Post-synthetic treatments (e.g., annealing temperature modifying crystallinity) .
Address these by replicating studies using identical parameters and publishing raw datasets for transparency .
Q. What advanced characterization techniques are critical for analyzing this compound’s electronic structure?
- X-ray absorption near-edge structure (XANES) : Probes the oxidation state and ligand environment of nickel .
- Extended X-ray absorption fine structure (EXAFS) : Quantifies bond lengths and coordination numbers around the metal center .
- Density functional theory (DFT) simulations : Validate experimental spectra and predict electronic localization effects .
Q. How can this compound be integrated into covalent organic frameworks (COFs) for enhanced functionality?
- Monomer design : Use octahydroxyphthalocyaninato nickel(II) [(OH)₈PcNi] with 1,4-benzenediboronic acid (BDBA) to form 2D COFs via solvothermal synthesis .
- Optimization : Adjust solvent systems (e.g., dimethylacetamide/o-dichlorobenzene) to improve crystallinity and surface area (>500 m²/g) .
- Applications : Leverage the ordered π-stacking in COFs for charge transport in photoconductive devices .
Q. What methodologies assess the thermal stability of this compound polymers under anaerobic conditions?
- Thermogravimetric analysis (TGA) : Measure weight loss profiles in nitrogen atmospheres; stable polymers retain >90% mass at 800°C .
- Char yield analysis : Correlate residual mass with crosslinking density and aromaticity .
- In situ FT-IR : Track decomposition pathways by identifying volatile byproducts .
Q. How can static and dynamic quenching mechanisms in this compound be distinguished experimentally?
- Time-resolved fluorescence : Dynamic quenching reduces excited-state lifetimes, while static quenching shows no lifetime change .
- Stern-Volmer analysis : Linear plots at low concentrations indicate dynamic quenching; upward curvature suggests static quenching .
- Temperature dependence : Static quenching decreases with temperature, while dynamic quenching increases .
Q. What strategies improve the catalytic selectivity of this compound in ethylene oligomerization?
- Ligand modification : Pyridine-imine substituents increase steric hindrance, favoring C₄ products (56.7% selectivity) over C₈ .
- Kinetic profiling : Monitor reaction rates using GC-MS and optimize Al/Ni molar ratios (e.g., 500:1) to suppress side reactions .
- Operando spectroscopy : Use UV-Vis to track active species and adjust pressure/temperature in real time .
Properties
CAS No. |
26893-94-7 |
---|---|
Molecular Formula |
C32H16N8Ni |
Molecular Weight |
571.2 g/mol |
IUPAC Name |
2,11,20,29,37,39-hexaza-38,40-diazanidanonacyclo[28.6.1.13,10.112,19.121,28.04,9.013,18.022,27.031,36]tetraconta-1,3,5,7,9,11,13,15,17,19(39),20,22,24,26,28,30(37),31,33,35-nonadecaene;nickel(2+) |
InChI |
InChI=1S/C32H16N8.Ni/c1-2-10-18-17(9-1)25-33-26(18)38-28-21-13-5-6-14-22(21)30(35-28)40-32-24-16-8-7-15-23(24)31(36-32)39-29-20-12-4-3-11-19(20)27(34-29)37-25;/h1-16H;/q-2;+2 |
InChI Key |
LVIYYTJTOKJJOC-UHFFFAOYSA-N |
Canonical SMILES |
C1=CC=C2C(=C1)C3=NC4=NC(=NC5=C6C=CC=CC6=C([N-]5)N=C7C8=CC=CC=C8C(=N7)N=C2[N-]3)C9=CC=CC=C94.[Ni+2] |
Related CAS |
26893-94-7 |
Origin of Product |
United States |
Disclaimer and Information on In-Vitro Research Products
Please be aware that all articles and product information presented on BenchChem are intended solely for informational purposes. The products available for purchase on BenchChem are specifically designed for in-vitro studies, which are conducted outside of living organisms. In-vitro studies, derived from the Latin term "in glass," involve experiments performed in controlled laboratory settings using cells or tissues. It is important to note that these products are not categorized as medicines or drugs, and they have not received approval from the FDA for the prevention, treatment, or cure of any medical condition, ailment, or disease. We must emphasize that any form of bodily introduction of these products into humans or animals is strictly prohibited by law. It is essential to adhere to these guidelines to ensure compliance with legal and ethical standards in research and experimentation.