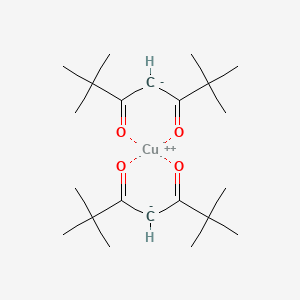
Cu(TMHD)2
- Click on QUICK INQUIRY to receive a quote from our team of experts.
- With the quality product at a COMPETITIVE price, you can focus more on your research.
Overview
Description
Copper bis(2,2,6,6-tetramethyl-3,5-heptanedionate), also known as copper bis(dipivaloylmethanato), is an organometallic compound with the molecular formula Cu(C_11H_19O_2)_2. This compound is characterized by its deep blue crystalline solid appearance and is stable at room temperature. It is soluble in organic solvents such as ether but insoluble in water .
Preparation Methods
Copper bis(2,2,6,6-tetramethyl-3,5-heptanedionate) is typically synthesized by reacting copper oxide with 2,2,6,6-tetramethyl-3,5-heptanedione in an organic solvent. There are several methods for this synthesis, including the solvent method and the solid-phase method . In one approach, copper acetate hydrate is reacted with 2,2,6,6-tetramethyl-3,5-heptanedione in a methanol solution . The reaction conditions, such as temperature and solvent choice, can significantly impact the yield and purity of the final product.
Chemical Reactions Analysis
Copper bis(2,2,6,6-tetramethyl-3,5-heptanedionate) undergoes various chemical reactions, including oxidation, reduction, and substitution reactions. It is commonly used in metal-organic chemical vapor deposition (MOCVD) processes to produce copper oxide thin films . The compound can be decomposed thermally, and its thermal stability and decomposition kinetics have been studied using thermogravimetric analysis (TGA) . The major products formed from these reactions include copper oxides such as cuprite (Cu_2O) and tenorite (CuO) .
Scientific Research Applications
Copper bis(2,2,6,6-tetramethyl-3,5-heptanedionate) has numerous scientific research applications. It is widely used in the field of materials science for the deposition of copper oxide thin films, which are essential in the development of electronic devices such as solar cells . The compound is also used as a catalyst in organic synthesis reactions and plays a significant role in oil refining and various chemical reactions . Additionally, it serves as a high-grade colorant and pigment in the paint and dye industries due to its deep blue color .
Mechanism of Action
The mechanism of action of copper bis(2,2,6,6-tetramethyl-3,5-heptanedionate) involves its decomposition and subsequent formation of copper oxides. During the MOCVD process, the compound is vaporized and transported to the substrate, where it decomposes to form a thin film of copper oxide . The thermal decomposition follows a phase boundary reaction mechanism, with an average activation energy of 85.1 kJ/mol .
Comparison with Similar Compounds
Copper bis(2,2,6,6-tetramethyl-3,5-heptanedionate) can be compared with other copper(II) precursors containing β-diketonate ligands, such as copper bis(2,2,7-trimethyl-3,5-octanedionate) and copper bis(2,2,6,6-tetramethyl-2-sila-3,5-heptanedionate) . These compounds are used in similar applications, such as chemical vapor deposition, but differ in their volatility and thermal stability. For example, copper bis(2,2,6,6-tetramethyl-2-sila-3,5-heptanedionate) is more volatile than copper bis(2,2,6,6-tetramethyl-3,5-heptanedionate), making it more suitable for certain deposition processes .
Biological Activity
Copper bis(2,2,6,6-tetramethyl-3,5-heptanedionate), commonly referred to as Cu(TMHD)₂, is a coordination compound that has garnered attention for its potential biological applications. This article explores the biological activity of Cu(TMHD)₂, highlighting its antibacterial properties, catalytic applications, and other relevant biological interactions based on diverse research findings.
1. Structural Characteristics of Cu(TMHD)₂
Cu(TMHD)₂ is characterized by its bidentate ligand structure, where the TMHD ligands coordinate with copper ions. The complex exhibits a typical tetrahedral geometry, which is crucial for its reactivity and interaction with biological systems. The stability and solubility of Cu(TMHD)₂ in various solvents make it an ideal candidate for further biological studies.
2. Antibacterial Properties
Recent studies have demonstrated that Cu(TMHD)₂ exhibits significant antibacterial activity against various bacterial strains. The mechanism of action appears to involve the generation of reactive oxygen species (ROS), which can damage bacterial cell membranes and DNA.
Case Study: Antibacterial Activity in Composite Coatings
A study investigated the incorporation of Cu(TMHD)₂ into titanium dioxide (TiO₂) composite coatings. The results indicated that increasing the mole fraction of Cu(TMHD)₂ enhanced the antibacterial properties of the coatings against both Gram-positive and Gram-negative bacteria. The antibacterial efficacy was attributed to the release of copper ions from the coating, which disrupted bacterial cellular functions .
Cu(TMHD)₂ Mole Fraction | Antibacterial Activity |
---|---|
0% | No activity |
1% | Moderate |
5% | High |
10% | Very High |
3. Catalytic Applications
Cu(TMHD)₂ serves as an effective catalyst in various organic reactions. Its catalytic properties have been explored in N-glycosylation reactions, where it has shown to facilitate the formation of glycosidic bonds efficiently.
Research Findings
In a study focusing on N-glycosylation reactions, Cu(TMHD)₂ was utilized as a catalyst alongside other ligands and bases. The results highlighted that Cu(TMHD)₂ achieved yields up to 66% for specific regioisomers without compromising selectivity . This demonstrates not only its catalytic efficiency but also its potential utility in synthetic organic chemistry.
4. Mechanistic Insights
The biological activity of Cu(TMHD)₂ can be attributed to several mechanisms:
- Reactive Oxygen Species Generation : The interaction of copper ions with cellular components leads to oxidative stress in bacteria.
- Enzyme Inhibition : Copper complexes can inhibit specific enzymes critical for bacterial survival.
- Membrane Disruption : Copper ions can integrate into bacterial membranes, altering permeability and leading to cell lysis.
5. Summary of Biological Activities
The following table summarizes key biological activities associated with Cu(TMHD)₂:
Activity Type | Description |
---|---|
Antibacterial | Effective against various bacterial strains |
Catalytic | Facilitates N-glycosylation with high yields |
Enzyme Inhibition | Potential inhibition of essential bacterial enzymes |
Oxidative Stress Induction | Induces ROS production leading to cellular damage |
Properties
Molecular Formula |
C22H38CuO4 |
---|---|
Molecular Weight |
430.1 g/mol |
IUPAC Name |
copper;2,2,6,6-tetramethylheptane-3,5-dione |
InChI |
InChI=1S/2C11H19O2.Cu/c2*1-10(2,3)8(12)7-9(13)11(4,5)6;/h2*7H,1-6H3;/q2*-1;+2 |
InChI Key |
JGMGCTZGOAONPQ-UHFFFAOYSA-N |
Canonical SMILES |
CC(C)(C)C(=O)[CH-]C(=O)C(C)(C)C.CC(C)(C)C(=O)[CH-]C(=O)C(C)(C)C.[Cu+2] |
Origin of Product |
United States |
Disclaimer and Information on In-Vitro Research Products
Please be aware that all articles and product information presented on BenchChem are intended solely for informational purposes. The products available for purchase on BenchChem are specifically designed for in-vitro studies, which are conducted outside of living organisms. In-vitro studies, derived from the Latin term "in glass," involve experiments performed in controlled laboratory settings using cells or tissues. It is important to note that these products are not categorized as medicines or drugs, and they have not received approval from the FDA for the prevention, treatment, or cure of any medical condition, ailment, or disease. We must emphasize that any form of bodily introduction of these products into humans or animals is strictly prohibited by law. It is essential to adhere to these guidelines to ensure compliance with legal and ethical standards in research and experimentation.