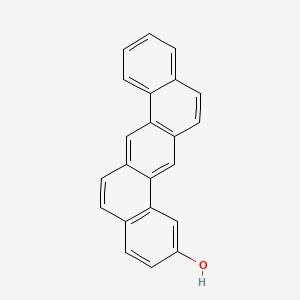
Dibenz(a,h)anthracen-2-ol
- Click on QUICK INQUIRY to receive a quote from our team of experts.
- With the quality product at a COMPETITIVE price, you can focus more on your research.
Overview
Description
Benzo[k]tetraphen-2-ol is an organic compound with the molecular formula C22H14O It is a polycyclic aromatic hydrocarbon (PAH) that consists of four fused benzene rings with a hydroxyl group attached to the second carbon atom
Preparation Methods
Synthetic Routes and Reaction Conditions
The synthesis of Benzo[k]tetraphen-2-ol typically involves transition metal-catalyzed kinetic ring annulation. One common method is the ruthenium-mediated aromatization, which requires multiple steps to achieve the desired product. Another approach involves the use of Yamamoto coupling, although this method has a low yield in the final step .
Industrial Production Methods
Industrial production of Benzo[k]tetraphen-2-ol is less common due to the complexity and low yield of the synthetic routes. advancements in synthetic chemistry may lead to more efficient production methods in the future.
Chemical Reactions Analysis
Types of Reactions
Benzo[k]tetraphen-2-ol undergoes various chemical reactions, including:
Oxidation: This reaction typically involves the use of oxidizing agents to convert the hydroxyl group into a carbonyl group.
Reduction: Reduction reactions can convert the hydroxyl group into a hydrogen atom, resulting in the formation of a hydrocarbon.
Substitution: Substitution reactions involve replacing the hydroxyl group with other functional groups, such as halogens or alkyl groups.
Common Reagents and Conditions
Oxidation: Common oxidizing agents include potassium permanganate (KMnO4) and chromium trioxide (CrO3).
Reduction: Reducing agents such as lithium aluminum hydride (LiAlH4) and sodium borohydride (NaBH4) are commonly used.
Substitution: Halogenation reactions often use reagents like bromine (Br2) or chlorine (Cl2) under specific conditions.
Major Products Formed
Oxidation: The major product is typically a ketone or aldehyde.
Reduction: The major product is a hydrocarbon.
Substitution: The major products are halogenated or alkylated derivatives of Benzo[k]tetraphen-2-ol.
Scientific Research Applications
Benzo[k]tetraphen-2-ol has several applications in scientific research, including:
Chemistry: It is used as a building block for the synthesis of more complex polycyclic aromatic hydrocarbons and other organic compounds.
Biology: Research on Benzo[k]tetraphen-2-ol includes its potential effects on biological systems, particularly its interactions with cellular components.
Medicine: The compound is studied for its potential therapeutic properties, including its role as an antioxidant or anticancer agent.
Mechanism of Action
The mechanism of action of Benzo[k]tetraphen-2-ol involves its interaction with molecular targets and pathways within biological systems. The hydroxyl group allows it to participate in hydrogen bonding and other interactions with proteins, nucleic acids, and other biomolecules. These interactions can influence cellular processes and pathways, leading to various biological effects.
Comparison with Similar Compounds
Benzo[k]tetraphen-2-ol is similar to other polycyclic aromatic hydrocarbons, such as:
Tetraphene: An angular ortho-fused polycyclic arene consisting of four fused benzene rings.
Benzo[a]anthracene: A polycyclic aromatic hydrocarbon with the chemical formula C18H12.
Uniqueness
The uniqueness of Benzo[k]tetraphen-2-ol lies in its specific structural arrangement and the presence of the hydroxyl group, which imparts distinct chemical and physical properties compared to other similar compounds.
Biological Activity
Dibenz(a,h)anthracen-2-ol, a polycyclic aromatic hydrocarbon (PAH), is known for its significant biological activity, particularly in terms of carcinogenicity and mutagenicity. This article explores its biological effects, mechanisms of action, and relevant case studies, supported by data tables and research findings.
Chemical Overview
This compound (DBAH) has the chemical formula C22H14 and is structurally related to dibenz[a,h]anthracene, a well-documented carcinogen. Its biological activity is primarily attributed to its ability to induce DNA damage and mutations in various organisms.
Carcinogenicity
DBAH is classified as a probable human carcinogen by multiple health organizations, including the International Agency for Research on Cancer (IARC). Studies have demonstrated that it can cause tumors in several animal models through various exposure routes.
Key Findings:
- Animal Studies: In mice, DBAH has been shown to induce mammary carcinomas and skin tumors following dermal application and oral administration. For example, a study indicated that a single dose of 1.5 mg resulted in forestomach papillomas in male Swiss mice after 30 weeks .
- DNA Damage: DBAH has been implicated in DNA adduct formation, which is a precursor to mutagenesis. In studies involving Muta™Mouse, DBAH exposure led to increased liver DNA adduct levels and lacZ mutant frequency .
The biological activity of DBAH is largely mediated by its metabolic activation. It undergoes biotransformation via cytochrome P450 enzymes, leading to the formation of reactive intermediates that can bind to DNA.
Metabolic Pathways:
- Activation: DBAH is metabolized into dihydrodiols that are mutagenic in bacterial assays. This metabolic pathway is similar to that of other PAHs like benzo[a]pyrene .
- Aryl Hydrocarbon Receptor (AhR) Activation: DBAH exhibits a higher potency for AhR activation compared to other PAHs, influencing gene expression related to carcinogenesis .
Biological Activity Data
The following table summarizes key studies on the biological activity of DBAH:
Study Reference | Exposure Route | Model Organism | Key Findings |
---|---|---|---|
Berenblum & Haran (1955) | Dermal | Swiss Mice | Induced skin tumors at concentrations ≥ 0.001% |
Biancifiori et al. (1962) | Oral | Balb/c Mice | Mammary carcinomas observed with doses as low as 15 mg/animal |
IARC Monograph (2010) | Various | Multiple Species | Positive evidence for carcinogenicity across different exposure routes |
EPA IRIS Assessment (1990) | Injection | Various Mouse Strains | Tumor incidence varied from 0% to 80% based on strain |
Case Studies
Several case studies have highlighted the implications of DBAH exposure in both laboratory settings and occupational environments.
Case Study 1: Occupational Exposure
In workers exposed to PAHs in industries such as aluminum production, elevated levels of DNA adducts were observed, correlating with increased cancer incidence. This supports the notion that DBAH contributes significantly to the carcinogenic risk associated with PAHs .
Case Study 2: Environmental Impact
Research has shown that DBAH can persist in contaminated soil and sediments, raising concerns about its long-term ecological impact and potential for human exposure through food chains .
Properties
CAS No. |
72007-85-3 |
---|---|
Molecular Formula |
C22H14O |
Molecular Weight |
294.3 g/mol |
IUPAC Name |
naphtho[1,2-b]phenanthren-2-ol |
InChI |
InChI=1S/C22H14O/c23-18-10-9-15-6-8-17-11-20-16(12-21(17)22(15)13-18)7-5-14-3-1-2-4-19(14)20/h1-13,23H |
InChI Key |
HSRPWTYLHBXRIB-UHFFFAOYSA-N |
Canonical SMILES |
C1=CC=C2C(=C1)C=CC3=C2C=C4C=CC5=C(C4=C3)C=C(C=C5)O |
Origin of Product |
United States |
Disclaimer and Information on In-Vitro Research Products
Please be aware that all articles and product information presented on BenchChem are intended solely for informational purposes. The products available for purchase on BenchChem are specifically designed for in-vitro studies, which are conducted outside of living organisms. In-vitro studies, derived from the Latin term "in glass," involve experiments performed in controlled laboratory settings using cells or tissues. It is important to note that these products are not categorized as medicines or drugs, and they have not received approval from the FDA for the prevention, treatment, or cure of any medical condition, ailment, or disease. We must emphasize that any form of bodily introduction of these products into humans or animals is strictly prohibited by law. It is essential to adhere to these guidelines to ensure compliance with legal and ethical standards in research and experimentation.