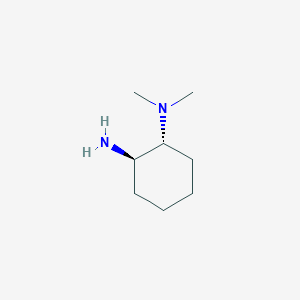
(1R,2R)-N1,N1-dimethylcyclohexane-1,2-diamine
Overview
Description
(1R,2R)-N1,N1-Dimethylcyclohexane-1,2-diamine (CAS: 67198-21-4) is a chiral cyclohexane diamine derivative with two methyl groups on the N1 nitrogen atom. Its stereochemistry, defined by the (1R,2R) configuration, plays a critical role in its applications in asymmetric catalysis, organocatalysis, and pharmaceutical precursor synthesis . The compound’s rigid cyclohexane backbone and dimethyl substitution pattern enhance its steric and electronic properties, making it a versatile scaffold for ligand design and bioactive molecule development .
Preparation Methods
Preparation Methods
Starting Materials and General Strategy
The synthesis of (1R,2R)-N1,N1-dimethylcyclohexane-1,2-diamine generally begins with enantiomerically pure (1R,2R)-cyclohexane-1,2-diamine or its derivatives. The key step involves selective N-methylation of the primary amino groups to introduce the N1,N1-dimethyl substitution while preserving the stereochemical integrity of the cyclohexane ring.
Synthetic Routes and Reaction Conditions
N-Methylation of (1R,2R)-Cyclohexane-1,2-diamine
- Reagents: Methyl iodide or dimethyl sulfate are commonly used methylating agents.
- Base: Sodium hydride or potassium carbonate serves as the base to deprotonate the amino groups.
- Solvent: Aprotic solvents such as tetrahydrofuran (THF) or dimethylformamide (DMF) are preferred.
- Conditions: The reaction is typically conducted at room temperature or slightly elevated temperatures to ensure selective mono- or dimethylation without overalkylation.
- Purification: The crude product is purified by recrystallization or column chromatography to achieve high purity and enantiomeric excess.
Alternative Multi-Step Synthesis via Carbamate Intermediates
- Starting from (R,R)-1,2-diammoniumcyclohexane mono-(+)-tartrate, treatment with ethyl chloroformate and sodium hydroxide forms diethyl-(1R,2R)-cyclohexane-1,2-diyldicarbamate.
- Subsequent reduction with lithium aluminum hydride (LiAlH4) yields the target diamine as a crystalline solid.
- This method requires strict control of stoichiometry and temperature to maintain enantiomeric purity.
Industrial Scale Production
- Continuous Flow Reactors: Industrial synthesis employs continuous flow reactors for better control over reaction parameters, improved safety, and scalability.
- Automation: Automated reagent addition and product separation enhance yield and reproducibility.
- Advantages: Continuous flow methods reduce reaction times and improve product consistency compared to batch processes.
Synthesis via Cyclohexene Oxide Route
An alternative synthetic approach involves:
- Reaction of cyclohexene oxide with aqueous methylamine (25–30%) at 80 °C for 5 hours to form trans-2-(methylamino)cyclohexanol.
- Mitsunobu reaction at room temperature followed by pH adjustment to yield 7-methyl-7-azabicyclo[4.1.0]heptane.
- Ring-opening of this intermediate with methylamine at 110 °C for 6 hours produces the target diamine without purification of intermediates.
Reaction Analysis and Characterization
Chemical Reactions Involved
Reaction Type | Reagents/Conditions | Products Formed |
---|---|---|
N-Methylation | Methyl iodide, NaH or K2CO3, THF/DMF, RT | This compound |
Carbamate Formation | Ethyl chloroformate, NaOH, aqueous medium | Diethyl-(1R,2R)-cyclohexane-1,2-diyldicarbamate |
Reduction | LiAlH4, anhydrous ether or THF | Target diamine |
Ring-Opening | Methylamine aqueous solution, 110 °C | Target diamine |
Purity and Stereochemical Confirmation
- NMR Spectroscopy: $$^{1}H$$ NMR shows characteristic signals for N–CH3 groups at δ ~2.33 ppm; $$^{13}C$$ NMR confirms methyl carbons at δ ~33.7 ppm.
- X-ray Crystallography: Confirms (1R,2R) stereochemistry with orthorhombic crystal system and intermolecular N–H···N hydrogen bonding stabilizing the lattice.
- Chiral HPLC and Polarimetry: Used to assess enantiomeric excess, typically achieving ≥94% purity.
- Gas Chromatography: Confirms chemical purity in the range of 94–98%.
Data Tables
Table 1: Key Physicochemical Properties
Table 2: Comparative Ligand Efficiency in Asymmetric Reactions
Ligand Derivative | Reaction Type | Enantiomeric Excess (ee) | Reference |
---|---|---|---|
(1R,2R)-N,N′-Diisobutyl | Ketone Alkylation | 92% | |
(1R,2R)-N-(Pyridyl-methyl) | Hg(II) Complex Formation | N/A (structural study) | |
Parent (1R,2R)-Diamine | Organolithium Deprotonation | 95% |
Chemical Reactions Analysis
Types of Reactions
(1R,2R)-N1,N1-dimethylcyclohexane-1,2-diamine undergoes various chemical reactions, including:
Oxidation: The compound can be oxidized using oxidizing agents like hydrogen peroxide or potassium permanganate to form corresponding imines or amides.
Reduction: Reduction reactions can be carried out using reducing agents such as lithium aluminum hydride or sodium borohydride to produce secondary amines.
Substitution: Nucleophilic substitution reactions can occur at the amino groups, leading to the formation of various substituted derivatives.
Common Reagents and Conditions
Oxidation: Hydrogen peroxide, potassium permanganate; typically carried out in aqueous or organic solvents at elevated temperatures.
Reduction: Lithium aluminum hydride, sodium borohydride; reactions are usually performed in anhydrous solvents like ether or tetrahydrofuran.
Substitution: Alkyl halides, acyl chlorides; reactions are conducted in the presence of a base such as triethylamine or pyridine.
Major Products Formed
Oxidation: Imines, amides
Reduction: Secondary amines
Substitution: N-alkylated or N-acylated derivatives
Scientific Research Applications
Asymmetric Synthesis and Catalysis
(1R,2R)-N1,N1-dimethylcyclohexane-1,2-diamine is extensively used as a chiral ligand in asymmetric synthesis. It plays a crucial role in catalyzing reactions that yield enantiomerically pure products, which are essential in pharmaceutical development where chirality can significantly influence drug efficacy and safety .
Biological Applications
The compound serves as a building block for synthesizing biologically active molecules. Its derivatives have been explored for potential applications in:
- Anticancer Agents: Research indicates that certain derivatives can target cancer cells effectively.
- Antimicrobial Compounds: The compound exhibits activity against various pathogens, making it valuable in developing new antibiotics .
Pharmaceutical Development
In medicinal chemistry, this compound is utilized to create drugs targeting specific enzymes and receptors. Its ability to selectively bind to biological targets allows for modulation of biochemical pathways, which is pivotal in drug design .
Industrial Applications
The compound is also used in the production of polymers and resins with enhanced mechanical and chemical properties. Its application extends to the manufacture of materials that require specific characteristics for industrial use .
Mechanism of Action
The mechanism by which (1R,2R)-N1,N1-dimethylcyclohexane-1,2-diamine exerts its effects involves its interaction with molecular targets such as enzymes and receptors. The chiral centers of the compound allow for specific binding to these targets, leading to the modulation of biochemical pathways. For example, in asymmetric catalysis, the compound acts as a chiral ligand, coordinating with metal centers to facilitate enantioselective reactions.
Comparison with Similar Compounds
Comparison with Structurally Similar Compounds
The following analysis compares (1R,2R)-N1,N1-dimethylcyclohexane-1,2-diamine with analogous diamines, focusing on structural variations, catalytic performance, pharmacological relevance, and physicochemical properties.
Structural and Substitution Variations
Key Observations :
- Steric Effects : Tetramethyl derivatives (e.g., L5 in ) exhibit higher steric hindrance, which can impede substrate binding in catalysis but improve enantioselectivity in certain reactions .
- Electronic Effects: Quinoline-substituted analogs (e.g., L4 in ) leverage aromatic π-systems for substrate activation, unlike the aliphatic dimethyl variant.
- Chirality Impact : Enantiomeric pairs (e.g., (1R,2R) vs. (1S,2S)) show divergent catalytic and pharmacological behaviors, as seen in opioid precursor studies .
Catalytic Activity
This compound serves as a ligand in asymmetric catalysis. For example:
- Mn(CO)₅Br-Catalyzed Hydrogenation: In isopropanol/methanol solvents, this diamine (L1) achieved 85–95% yield in aromatic ketone hydrogenation, outperforming non-methylated analogs (L3) due to improved electron-donating methyl groups .
- Organocatalysis: Derivatives of (1R,2R)-cyclohexane-1,2-diamine with benzenediamine H-bond donors achieved up to 93% conversion in Michael additions, though enantioselectivity remained moderate (41% ee) .
Comparison with Tetramethyl Analogs :
- The tetramethyl derivative (L5 in ) showed reduced catalytic efficiency in hydrogenation, likely due to excessive steric bulk, but improved stereochemical control in epoxidation reactions .
Pharmacological Relevance
- Antiviral Potential: The dimethyl variant was identified as a safer analog of chloroquine derivatives, interacting with SARS-CoV-2 spike protein with reduced cytotoxicity .
- Opioid Precursors : this compound is a precursor to U-series synthetic opioids, with methylation influencing receptor binding affinity .
Physicochemical Properties
Property | This compound | (1R,2R)-Tetramethyl Analogue | Parent Diamine (L3) |
---|---|---|---|
Molecular Weight (g/mol) | 156.27 | 184.32 | 128.21 |
Purity (Commercial) | 97% | >95% | >98% |
Solubility | High in polar solvents (e.g., MeOH, iPrOH) | Moderate in THF | High in H₂O |
Biological Activity
Overview
(1R,2R)-N1,N1-dimethylcyclohexane-1,2-diamine is a chiral diamine with significant applications in organic chemistry and biological research. Its unique structure, characterized by two chiral centers, allows it to interact selectively with various biological targets, influencing biochemical pathways and cellular functions.
- Molecular Formula: C8H18N2
- Molecular Weight: 158.25 g/mol
- CAS Number: 67198-21-4
- Appearance: White to light yellow crystalline powder
The biological activity of this compound is primarily attributed to its ability to act as a ligand in asymmetric synthesis and catalysis. It interacts with various enzymes and receptors due to its chiral nature, leading to modulation of biochemical pathways. Notable mechanisms include:
- Enzyme Interaction: The compound can inhibit or activate specific enzymes, impacting metabolic pathways.
- Receptor Binding: Its structural configuration allows for selective binding to receptors, influencing signal transduction processes.
1. Asymmetric Synthesis
This compound is extensively used as a chiral ligand in the synthesis of enantiomerically pure compounds. This application is crucial in pharmaceutical development where chirality can significantly affect drug efficacy and safety.
2. Pharmaceutical Development
The compound serves as a building block for synthesizing biologically active molecules, including:
- Anticancer Agents: Its derivatives have been explored for their potential in targeting cancer cells.
- Antimicrobial Compounds: Research indicates activity against various pathogens.
3. Agrochemicals
In agricultural chemistry, it is utilized in developing pesticides and herbicides that require specific molecular configurations for effectiveness.
Study 1: Enzyme Inhibition
A study investigated the inhibitory effects of this compound on a specific enzyme involved in metabolic processes. The results demonstrated a significant reduction in enzyme activity at concentrations above 10 μM, suggesting potential applications in metabolic regulation.
Study 2: Cellular Effects
In vitro studies on cell lines revealed that treatment with this compound led to alterations in gene expression related to apoptosis and cell proliferation. This indicates its potential role in cancer therapeutics.
Research Findings
Study | Focus | Findings |
---|---|---|
Strohmann et al., 2008 | Enzyme Interaction | Demonstrated selective inhibition of metabolic enzymes |
PMC2977656 | Anticancer Activity | Identified potential cytotoxic effects on cancer cell lines |
Chemical Book | Synthesis Applications | Highlighted use as a ligand in various coupling reactions |
Q & A
Basic Research Questions
Q. What are the optimal synthetic routes for enantiomerically pure (1R,2R)-N1,N1-dimethylcyclohexane-1,2-diamine?
The compound is synthesized via a two-step process starting from (R,R)-1,2-diammoniumcyclohexane mono-(+)-tartrate. First, treatment with ethyl chloroformate and NaOH forms diethyl-(1R,2R)-cyclohexane-1,2-diyldicarbamate. Subsequent reduction with LiAlH4 yields the title compound as a crystalline solid. Key challenges include maintaining enantiomeric purity, which requires strict control of reaction conditions (e.g., stoichiometry and temperature). NMR (¹H: δ 2.33 ppm for N–CH₃; ¹³C: δ 33.7 ppm for CH₃) confirms structural integrity .
Q. How can researchers confirm the stereochemical configuration and purity of this diamine?
X-ray crystallography is the gold standard for confirming stereochemistry. The compound crystallizes in the orthorhombic system (space group P2₁2₁2₁) with intermolecular N–H···N hydrogen bonds (3.25 Å distance) stabilizing the lattice . Chiral HPLC or polarimetry can assess enantiomeric excess, while GC (≥94–98% purity) evaluates chemical purity .
Q. What safety protocols are critical when handling this compound?
The diamine is hygroscopic and air-sensitive, requiring storage under inert atmospheres. Hazard codes H315 (skin irritation), H319 (eye irritation), and H335 (respiratory irritation) mandate the use of gloves, goggles, and fume hoods. Precautionary measures (P261, P305+P351+P338) include avoiding inhalation and immediate rinsing upon contact .
Advanced Research Questions
Q. How does the C2-symmetric structure of this diamine influence its coordination chemistry with transition metals?
The (1R,2R) configuration enables chelation of metals like Hg(II) and Zn(II), forming complexes with distorted tetrahedral or octahedral geometries. For example, in dichlorido[(1R,2R)-N-(pyridin-2-yl-methyl)cyclohexane-1,2-diamine]mercury(II), the ligand adopts a tridentate (N,N′,N′′) coordination mode, enhancing stereoselectivity in catalytic reactions . Comparative studies show asymmetric N-substituents (e.g., isobutyl groups) improve reaction efficiency over symmetric analogs .
Q. What role does this diamine play in asymmetric organolithium reactions?
As a chiral ligand, it deaggregates organolithium reagents (e.g., LDA), increasing their nucleophilicity and stereocontrol. For instance, in deprotonation reactions, the diamine’s rigid cyclohexane backbone directs substrate approach, achieving high enantiomeric ratios (e.g., >95:5 in ketone alkylations). Mechanistic studies highlight hydrogen bonding between NH groups and lithiated intermediates as critical for selectivity .
Q. How do intermolecular interactions in the crystalline phase affect its physical properties?
Weak N–H···N hydrogen bonds (N···N: 3.250 Å) create layered structures, influencing melting behavior (45–47°C) and solubility. Polymorphic variations, such as protonation states (e.g., NH₂⁺ in salts), alter melting points and hygroscopicity. Differential scanning calorimetry (DSC) can identify phase transitions linked to these interactions .
Q. What strategies resolve contradictions in reported CAS numbers and molecular formulas?
Q. How can computational modeling predict ligand-metal binding affinities?
Density Functional Theory (DFT) calculations (e.g., B3LYP/6-31G*) optimize geometries and calculate binding energies. For example, the diamine’s N–Hg bond lengths in mercury complexes (~2.3–2.5 Å) align with crystallographic data, validating models .
Q. Tables
Table 1: Key Physicochemical Properties
Property | Value | Reference |
---|---|---|
Molecular Formula | C₈H₁₈N₂ (neutral) / C₈H₂₀N₂ (protonated) | |
Melting Point | 45–47°C | |
Enantiomeric Purity | ≥94% (GC) | |
Hazard Codes | H315, H319, H335 |
Table 2: Comparative Ligand Efficiency in Asymmetric Reactions
Properties
IUPAC Name |
(1R,2R)-2-N,2-N-dimethylcyclohexane-1,2-diamine | |
---|---|---|
Source | PubChem | |
URL | https://pubchem.ncbi.nlm.nih.gov | |
Description | Data deposited in or computed by PubChem | |
InChI |
InChI=1S/C8H18N2/c1-10(2)8-6-4-3-5-7(8)9/h7-8H,3-6,9H2,1-2H3/t7-,8-/m1/s1 | |
Source | PubChem | |
URL | https://pubchem.ncbi.nlm.nih.gov | |
Description | Data deposited in or computed by PubChem | |
InChI Key |
FRDZGSBXKJXGNR-HTQZYQBOSA-N | |
Source | PubChem | |
URL | https://pubchem.ncbi.nlm.nih.gov | |
Description | Data deposited in or computed by PubChem | |
Canonical SMILES |
CN(C)C1CCCCC1N | |
Source | PubChem | |
URL | https://pubchem.ncbi.nlm.nih.gov | |
Description | Data deposited in or computed by PubChem | |
Isomeric SMILES |
CN(C)[C@@H]1CCCC[C@H]1N | |
Source | PubChem | |
URL | https://pubchem.ncbi.nlm.nih.gov | |
Description | Data deposited in or computed by PubChem | |
Molecular Formula |
C8H18N2 | |
Source | PubChem | |
URL | https://pubchem.ncbi.nlm.nih.gov | |
Description | Data deposited in or computed by PubChem | |
DSSTOX Substance ID |
DTXSID30460779 | |
Record name | Trans-N,N-Dimethylcyclohexane-1,2-Diamine | |
Source | EPA DSSTox | |
URL | https://comptox.epa.gov/dashboard/DTXSID30460779 | |
Description | DSSTox provides a high quality public chemistry resource for supporting improved predictive toxicology. | |
Molecular Weight |
142.24 g/mol | |
Source | PubChem | |
URL | https://pubchem.ncbi.nlm.nih.gov | |
Description | Data deposited in or computed by PubChem | |
CAS No. |
67198-21-4, 320778-92-5 | |
Record name | Trans-N,N-Dimethylcyclohexane-1,2-Diamine | |
Source | EPA DSSTox | |
URL | https://comptox.epa.gov/dashboard/DTXSID30460779 | |
Description | DSSTox provides a high quality public chemistry resource for supporting improved predictive toxicology. | |
Record name | (1R,2R)-N1,N1-Dimethylcyclohexane-1,2-diamine | |
Source | European Chemicals Agency (ECHA) | |
URL | https://echa.europa.eu/information-on-chemicals | |
Description | The European Chemicals Agency (ECHA) is an agency of the European Union which is the driving force among regulatory authorities in implementing the EU's groundbreaking chemicals legislation for the benefit of human health and the environment as well as for innovation and competitiveness. | |
Explanation | Use of the information, documents and data from the ECHA website is subject to the terms and conditions of this Legal Notice, and subject to other binding limitations provided for under applicable law, the information, documents and data made available on the ECHA website may be reproduced, distributed and/or used, totally or in part, for non-commercial purposes provided that ECHA is acknowledged as the source: "Source: European Chemicals Agency, http://echa.europa.eu/". Such acknowledgement must be included in each copy of the material. ECHA permits and encourages organisations and individuals to create links to the ECHA website under the following cumulative conditions: Links can only be made to webpages that provide a link to the Legal Notice page. | |
Retrosynthesis Analysis
AI-Powered Synthesis Planning: Our tool employs the Template_relevance Pistachio, Template_relevance Bkms_metabolic, Template_relevance Pistachio_ringbreaker, Template_relevance Reaxys, Template_relevance Reaxys_biocatalysis model, leveraging a vast database of chemical reactions to predict feasible synthetic routes.
One-Step Synthesis Focus: Specifically designed for one-step synthesis, it provides concise and direct routes for your target compounds, streamlining the synthesis process.
Accurate Predictions: Utilizing the extensive PISTACHIO, BKMS_METABOLIC, PISTACHIO_RINGBREAKER, REAXYS, REAXYS_BIOCATALYSIS database, our tool offers high-accuracy predictions, reflecting the latest in chemical research and data.
Strategy Settings
Precursor scoring | Relevance Heuristic |
---|---|
Min. plausibility | 0.01 |
Model | Template_relevance |
Template Set | Pistachio/Bkms_metabolic/Pistachio_ringbreaker/Reaxys/Reaxys_biocatalysis |
Top-N result to add to graph | 6 |
Feasible Synthetic Routes
Disclaimer and Information on In-Vitro Research Products
Please be aware that all articles and product information presented on BenchChem are intended solely for informational purposes. The products available for purchase on BenchChem are specifically designed for in-vitro studies, which are conducted outside of living organisms. In-vitro studies, derived from the Latin term "in glass," involve experiments performed in controlled laboratory settings using cells or tissues. It is important to note that these products are not categorized as medicines or drugs, and they have not received approval from the FDA for the prevention, treatment, or cure of any medical condition, ailment, or disease. We must emphasize that any form of bodily introduction of these products into humans or animals is strictly prohibited by law. It is essential to adhere to these guidelines to ensure compliance with legal and ethical standards in research and experimentation.