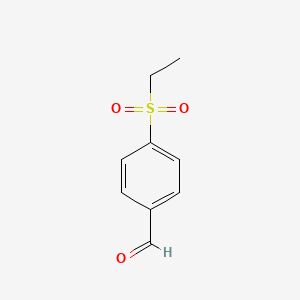
4-Ethylsulfonylbenzaldehyde
Overview
Description
4-Ethylsulfonylbenzaldehyde is a chemical compound with the molecular formula C9H10O3S and a molecular weight of 198.24 . It is used for research and development purposes .
Molecular Structure Analysis
The molecular structure of 4-Ethylsulfonylbenzaldehyde consists of 9 carbon atoms, 10 hydrogen atoms, 3 oxygen atoms, and 1 sulfur atom . For a detailed structural analysis, please refer to a reliable chemical database or a chemistry professional.Physical And Chemical Properties Analysis
4-Ethylsulfonylbenzaldehyde is a solid substance . It has a melting point of 108-110°C and a boiling point of 384.41°C at 760 mmHg. Its density is predicted to be 1.24 g/cm³ and its refractive index is predicted to be n20D 1.54 .Scientific Research Applications
1. Analytical Chemistry and Colorimetric Assays
4-Ethylsulfonylbenzaldehyde derivatives have been used in analytical chemistry, particularly in the development of colorimetric assays. For example, specific reactions under acidic conditions involving related aldehydes can yield chromophores with intense maximal absorbance, useful in lipid peroxidation assays (Gérard-Monnier et al., 1998).
2. Catalysis and Organic Synthesis
In organic synthesis, derivatives of 4-Ethylsulfonylbenzaldehyde have been used as catalysts or intermediates. For instance, certain aldehyde derivatives are involved in oxyfunctionalization reactions under ligand- and additive-free conditions, indicating their potential as catalysts or reactants in synthesizing aromatic carbonyl compounds (Jiang et al., 2014).
3. Solubility and Thermodynamics Studies
The solubility and solution thermodynamics of similar aldehydes in various organic solvents have been studied, providing essential data for understanding their behavior in different mediums. Such information is crucial for designing purification processes and optimizing reaction conditions (Wang et al., 2017).
4. Material Science and Polymer Chemistry
In material science, aldehydes structurally related to 4-Ethylsulfonylbenzaldehyde have been investigated for their potential in synthesizing polymers and as components in materials with specific properties (Temprado et al., 2008). This includes the study of their thermophysical properties and applications in solid-phase organic synthesis.
properties
IUPAC Name |
4-ethylsulfonylbenzaldehyde | |
---|---|---|
Source | PubChem | |
URL | https://pubchem.ncbi.nlm.nih.gov | |
Description | Data deposited in or computed by PubChem | |
InChI |
InChI=1S/C9H10O3S/c1-2-13(11,12)9-5-3-8(7-10)4-6-9/h3-7H,2H2,1H3 | |
Source | PubChem | |
URL | https://pubchem.ncbi.nlm.nih.gov | |
Description | Data deposited in or computed by PubChem | |
InChI Key |
ZAYJHTQPWWNPHO-UHFFFAOYSA-N | |
Source | PubChem | |
URL | https://pubchem.ncbi.nlm.nih.gov | |
Description | Data deposited in or computed by PubChem | |
Canonical SMILES |
CCS(=O)(=O)C1=CC=C(C=C1)C=O | |
Source | PubChem | |
URL | https://pubchem.ncbi.nlm.nih.gov | |
Description | Data deposited in or computed by PubChem | |
Molecular Formula |
C9H10O3S | |
Source | PubChem | |
URL | https://pubchem.ncbi.nlm.nih.gov | |
Description | Data deposited in or computed by PubChem | |
DSSTOX Substance ID |
DTXSID20528751 | |
Record name | 4-(Ethanesulfonyl)benzaldehyde | |
Source | EPA DSSTox | |
URL | https://comptox.epa.gov/dashboard/DTXSID20528751 | |
Description | DSSTox provides a high quality public chemistry resource for supporting improved predictive toxicology. | |
Molecular Weight |
198.24 g/mol | |
Source | PubChem | |
URL | https://pubchem.ncbi.nlm.nih.gov | |
Description | Data deposited in or computed by PubChem | |
Product Name |
4-Ethylsulfonylbenzaldehyde | |
CAS RN |
50899-03-1 | |
Record name | 4-(Ethanesulfonyl)benzaldehyde | |
Source | EPA DSSTox | |
URL | https://comptox.epa.gov/dashboard/DTXSID20528751 | |
Description | DSSTox provides a high quality public chemistry resource for supporting improved predictive toxicology. | |
Retrosynthesis Analysis
AI-Powered Synthesis Planning: Our tool employs the Template_relevance Pistachio, Template_relevance Bkms_metabolic, Template_relevance Pistachio_ringbreaker, Template_relevance Reaxys, Template_relevance Reaxys_biocatalysis model, leveraging a vast database of chemical reactions to predict feasible synthetic routes.
One-Step Synthesis Focus: Specifically designed for one-step synthesis, it provides concise and direct routes for your target compounds, streamlining the synthesis process.
Accurate Predictions: Utilizing the extensive PISTACHIO, BKMS_METABOLIC, PISTACHIO_RINGBREAKER, REAXYS, REAXYS_BIOCATALYSIS database, our tool offers high-accuracy predictions, reflecting the latest in chemical research and data.
Strategy Settings
Precursor scoring | Relevance Heuristic |
---|---|
Min. plausibility | 0.01 |
Model | Template_relevance |
Template Set | Pistachio/Bkms_metabolic/Pistachio_ringbreaker/Reaxys/Reaxys_biocatalysis |
Top-N result to add to graph | 6 |
Feasible Synthetic Routes
Disclaimer and Information on In-Vitro Research Products
Please be aware that all articles and product information presented on BenchChem are intended solely for informational purposes. The products available for purchase on BenchChem are specifically designed for in-vitro studies, which are conducted outside of living organisms. In-vitro studies, derived from the Latin term "in glass," involve experiments performed in controlled laboratory settings using cells or tissues. It is important to note that these products are not categorized as medicines or drugs, and they have not received approval from the FDA for the prevention, treatment, or cure of any medical condition, ailment, or disease. We must emphasize that any form of bodily introduction of these products into humans or animals is strictly prohibited by law. It is essential to adhere to these guidelines to ensure compliance with legal and ethical standards in research and experimentation.