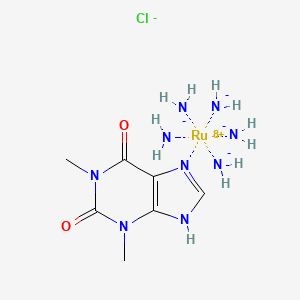
azanide;1,3-dimethyl-9H-purine-2,6-dione;ruthenium(8+);chloride
- Click on QUICK INQUIRY to receive a quote from our team of experts.
- With the quality product at a COMPETITIVE price, you can focus more on your research.
Overview
Description
This compound comprises a ruthenium center in the rare +8 oxidation state, coordinated with azanide (NH₂⁻), 1,3-dimethyl-9H-purine-2,6-dione (a methylated xanthine derivative), and chloride counterions.
Preparation Methods
Synthetic Routes and Reaction Conditions
The synthesis of azanide;1,3-dimethyl-9H-purine-2,6-dione;ruthenium(8+);chloride typically involves the coordination of ruthenium with theophylline and azanide. One common method involves dissolving theophylline in ethanol and adding ruthenium chloride under controlled conditions. The reaction mixture is then stirred and heated to facilitate the formation of the complex .
Industrial Production Methods
Industrial production of this compound may involve similar synthetic routes but on a larger scale. The process would include precise control of reaction conditions such as temperature, pressure, and pH to ensure high yield and purity of the final product. Advanced techniques like crystallization and chromatography may be employed for purification .
Chemical Reactions Analysis
Types of Reactions
Azanide;1,3-dimethyl-9H-purine-2,6-dione;ruthenium(8+);chloride can undergo various chemical reactions, including:
Oxidation: The ruthenium center can participate in oxidation reactions, potentially altering the oxidation state of the metal.
Reduction: The compound can also undergo reduction reactions, where the ruthenium center gains electrons.
Substitution: Ligands in the complex can be substituted with other ligands under appropriate conditions.
Common Reagents and Conditions
Common reagents used in these reactions include oxidizing agents like hydrogen peroxide for oxidation, reducing agents like sodium borohydride for reduction, and various ligands for substitution reactions. The reactions typically require controlled temperatures and inert atmospheres to prevent unwanted side reactions .
Major Products Formed
The major products formed from these reactions depend on the specific reagents and conditions used. For example, oxidation may yield higher oxidation state ruthenium complexes, while reduction may produce lower oxidation state complexes. Substitution reactions can result in new complexes with different ligands .
Scientific Research Applications
It appears that the query is asking for information on the applications of the chemical compound "azanide;1,3-dimethyl-9H-purine-2,6-dione;ruthenium(8+);chloride." However, the search results provide limited information about the applications of this specific compound. The available data primarily focuses on its chemical properties, related compounds, and structural identifiers.
Here's what can be gathered from the search results:
Chemical Information
- Structure: The compound contains azanide, 1,3-dimethyl-9H-purine-2,6-dione, ruthenium(8+), and chloride .
- PubChem: PubChem provides access to chemical information, allowing searches by name, molecular formula, structure, and other identifiers . It offers chemical and physical properties, biological activities, safety and toxicity information, patents, and literature citations .
- CAS Registry Number: One search result lists CAS No. 55208-38-3 for a related compound, "azane, 1,3-dimethyl-7H-purine-2,6-dione, ruthenium(+3) cation, trichlo ride" .
- ChemicalBook: Mentions several chemicals, including "4-Azaisatin" and other azane derivatives .
Related Compounds
- 1,3-Dimethyl-8-nitro-3,7-dihydro-1H-purine-2,6-dione: PubChem provides detailed information on this compound, including its molecular formula (C7H7N5O4), molecular weight (225.16 g/mol), IUPAC name, InChI, InChIKey, and SMILES notation .
- Pyrene: This compound is listed in the DETHERM database directory with various combinations .
Mechanism of Action
The mechanism of action of azanide;1,3-dimethyl-9H-purine-2,6-dione;ruthenium(8+);chloride involves the interaction of the ruthenium center with various molecular targets. In biological systems, it can bind to DNA and proteins, disrupting their function. The compound may also inhibit certain enzymes by binding to their active sites, thereby blocking their activity. The exact pathways involved depend on the specific application and target molecules .
Comparison with Similar Compounds
Ruthenium Chloride Hydrates
Ruthenium(III) chloride hydrates (RuCl₃·nH₂O) are widely studied precursors for catalysis and materials science. Key differences include:
Polynuclear ruthenium complexes, such as [Ru₈(p-cymene)₈(tpp-H₂)₂(oxalato)₄]⁸⁺, exhibit lower cytotoxicity compared to smaller clusters, suggesting that nuclearity inversely correlates with biological activity .
Azanide-Containing Metal Complexes
Mercuric azanide chloride (HgNH₂Cl) shares the NH₂⁻ ligand but differs markedly:
Property | Azanide-Purine-Ru(8+) Complex | HgNH₂Cl |
---|---|---|
Toxicity | Likely lower | Highly toxic |
Structure | Polynuclear Ru cluster | Mononuclear Hg complex |
Applications | Biomedical research | Limited (historical use) |
Purine-Ligand Metal Complexes
Cisplatin (Cl₂H₄N₂Pt) and similar platinum complexes are benchmark anticancer agents. Comparatively:
Biological Activity
The compound azanide; 1,3-dimethyl-9H-purine-2,6-dione; ruthenium(8+); chloride represents a class of metal complexes that have garnered attention for their potential biological activities, particularly in the fields of oncology and neuroprotection. Ruthenium-based compounds are being studied as alternatives to traditional platinum-based drugs due to their unique properties, including lower toxicity and enhanced selectivity toward cancer cells.
Ruthenium complexes exhibit various mechanisms of action that contribute to their biological activity:
- Anticancer Activity : Ruthenium compounds can induce apoptosis in cancer cells through the generation of reactive oxygen species (ROS), which leads to oxidative stress and subsequent cell death. The interaction with cellular components such as DNA and proteins plays a crucial role in this process .
- Neuroprotective Effects : Certain ruthenium complexes have shown promise in protecting neuronal cells from toxic agents, such as β-amyloid aggregates associated with Alzheimer's disease. This is achieved by inhibiting the aggregation of amyloid peptides and scavenging their toxic effects .
In Vitro Studies
-
Cytotoxicity Against Cancer Cells :
- Various studies have demonstrated that ruthenium complexes possess significant cytotoxic effects against a range of cancer cell lines. For instance, compounds derived from azanide showed lower IC50 values compared to traditional chemotherapeutics like cisplatin, indicating higher potency against specific cancer types .
- Table 1 summarizes the cytotoxicity data for selected ruthenium complexes:
Compound IC50 (µM) Cancer Cell Line Azanide-Ruthenium Complex 30 A2780 (Ovarian Cancer) PMRU20 25 Cortical Neurons KP1019 50 HCT116 (Colon Cancer) - Neuroprotective Activity :
In Vivo Studies
In vivo experiments using zebrafish models have shown that certain ruthenium complexes do not exhibit significant systemic toxicity while maintaining anticancer efficacy. For example, JHOR11 was effective against colon cancer xenografts without notable side effects .
Case Studies
-
Ruthenium Complexes in Cancer Therapy :
- A study investigating the effects of various ruthenium complexes on human ovarian carcinoma cells highlighted their ability to overcome multidrug resistance (MDR). The complexes demonstrated selective cytotoxicity towards cancer cells while sparing non-tumorigenic cell lines, suggesting a favorable therapeutic index .
- Neuroprotection Against Alzheimer’s Disease :
Q & A
Q. Basic: What are the optimal synthetic routes for preparing ruthenium(III) chloride complexes with azanide and 1,3-dimethyl-9H-purine-2,6-dione ligands?
Methodological Answer:
Ruthenium(III) chloride (RuCl₃) is typically synthesized via direct chlorination of ruthenium metal or reduction of RuO₄ in HCl. For coordination with azanide (NH₂⁻) and 1,3-dimethyl-9H-purine-2,6-dione (a xanthine derivative), the following steps are recommended:
Ligand Preparation : Purify 1,3-dimethyl-9H-purine-2,6-dione via recrystallization (e.g., using ethanol/water mixtures) to ensure ligand integrity.
Coordination Reaction : Reflux RuCl₃·xH₂O with the ligand in anhydrous methanol or dichloromethane under inert atmosphere (N₂/Ar) for 6–12 hours.
Precipitation : Add diethyl ether to precipitate the complex, followed by vacuum filtration.
Characterization : Confirm structure via IR (amide N–H stretches ~3300 cm⁻¹), ¹H/³¹P NMR (ligand proton environments), and elemental analysis .
Key Considerations :
- Hydrate vs. anhydrous RuCl₃: Hydrated forms (e.g., RuCl₃·3H₂O) may alter reaction kinetics due to water ligands .
- Ligand-to-metal ratio: Optimize stoichiometry (e.g., 2:1 ligand:Ru) to avoid byproducts.
Q. Advanced: How do electronic properties of 1,3-dimethyl-9H-purine-2,6-dione influence the catalytic activity of RuCl₃ complexes?
Methodological Answer :
The ligand’s electron-donating/withdrawing groups modulate Ru(III)’s redox behavior. For example:
- Electron Density Analysis : Use natural bond orbital (NBO) analysis to quantify charge transfer from ligand lone pairs (e.g., purine N atoms) to Ru³⁺. Lower electron density at Ru enhances oxidative catalysis .
- Catalytic Applications :
- Organic Transformations : RuCl₃ complexes catalyze C–H activation in arenes (e.g., benzene to [RuCl₂(C₆H₆)]₂ via cyclohexadiene intermediates ).
- Photophysical Studies : TD-DFT calculations reveal ligand-to-metal charge transfer (LMCT) transitions in UV-Vis spectra (~450–600 nm), critical for photocatalysis .
Methodological Answer :
IR Spectroscopy : Identify NH₂⁻ (azanide) stretches (~1600–1650 cm⁻¹) and Ru–Cl vibrations (250–350 cm⁻¹) .
NMR :
- ¹H NMR: Resolve purine methyl groups (δ 3.2–3.5 ppm) and aromatic protons (δ 7.0–8.5 ppm).
- ³¹P NMR (if phosphine co-ligands): Detect Ru–P coupling constants (~50–100 Hz) .
X-ray Crystallography : Resolve Ru–N (azanide) and Ru–O (purine) bond lengths (typically 2.0–2.2 Å) .
Example Workflow :
- Sample Prep : Grow single crystals via slow diffusion of ether into acetonitrile solutions.
- Data Collection : Use synchrotron radiation for high-resolution structures (CCDC deposition recommended) .
Q. Advanced: How can computational methods resolve contradictions in reported RuCl₃ complex properties?
Methodological Answer :
Discrepancies in decomposition temperatures (e.g., anhydrous RuCl₃ >500°C vs. hydrate ~100°C ) arise from hydration state and ligand effects.
DFT Optimization : Compare gas-phase vs. solvated models to assess hydration energy.
Thermogravimetric Analysis (TGA) : Correlate experimental mass loss (e.g., 18% for RuCl₃·3H₂O) with computed dehydration pathways .
Reaction Mechanism Studies : Use QM/MM to model ligand substitution kinetics (e.g., H₂O → purine in aqueous media) .
Q. Basic: What safety protocols are critical when handling azanide and RuCl₃ complexes?
Methodological Answer :
Azanide (NH₂⁻) : Store under inert atmosphere (glovebox) due to high reactivity with moisture/CO₂.
RuCl₃ : Use fume hoods; hydrate forms are hygroscopic and may release HCl vapors upon decomposition .
Waste Disposal : Neutralize acidic byproducts with NaHCO₃ before aqueous disposal .
Q. Advanced: How do solvent choices impact the stability of Ru-purine complexes?
Methodological Answer :
- Polar Aprotic Solvents (DMF, DMSO) : Stabilize Ru(III) via strong coordination but may displace labile ligands.
- Chlorinated Solvents (CH₂Cl₂) : Ideal for inert reactions but risk Cl⁻ ligand competition.
- Water : Limited use due to hydrolysis (e.g., [RuCl₂(H₂O)₄]⁺ formation). Validate stability via cyclic voltammetry (Ru³⁺/Ru²⁺ redox peaks) .
Q. Data Contradiction Analysis: Why do different studies report varying solubility for RuCl₃ complexes?
Resolution Strategy :
Properties
Molecular Formula |
C7H18ClN9O2Ru+2 |
---|---|
Molecular Weight |
396.8 g/mol |
IUPAC Name |
azanide;1,3-dimethyl-9H-purine-2,6-dione;ruthenium(8+);chloride |
InChI |
InChI=1S/C7H8N4O2.ClH.5H2N.Ru/c1-10-5-4(8-3-9-5)6(12)11(2)7(10)13;;;;;;;/h3H,1-2H3,(H,8,9);1H;5*1H2;/q;;5*-1;+8/p-1 |
InChI Key |
NOZKLQIVUAGQBZ-UHFFFAOYSA-M |
Canonical SMILES |
CN1C2=C(C(=O)N(C1=O)C)N=CN2.[NH2-].[NH2-].[NH2-].[NH2-].[NH2-].[Cl-].[Ru+8] |
Origin of Product |
United States |
Disclaimer and Information on In-Vitro Research Products
Please be aware that all articles and product information presented on BenchChem are intended solely for informational purposes. The products available for purchase on BenchChem are specifically designed for in-vitro studies, which are conducted outside of living organisms. In-vitro studies, derived from the Latin term "in glass," involve experiments performed in controlled laboratory settings using cells or tissues. It is important to note that these products are not categorized as medicines or drugs, and they have not received approval from the FDA for the prevention, treatment, or cure of any medical condition, ailment, or disease. We must emphasize that any form of bodily introduction of these products into humans or animals is strictly prohibited by law. It is essential to adhere to these guidelines to ensure compliance with legal and ethical standards in research and experimentation.