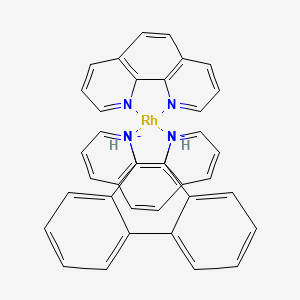
Bis(9,10-phenanthrenequinonediimine)(phenanthroline)rhodium(III)
- Click on QUICK INQUIRY to receive a quote from our team of experts.
- With the quality product at a COMPETITIVE price, you can focus more on your research.
Overview
Description
Bis(9,10-phenanthrenequinonediimine)(phenanthroline)rhodium(III) is a coordination compound that has garnered significant interest in the field of chemistry due to its unique structural and electronic properties This compound features a rhodium(III) center coordinated with two 9,10-phenanthrenequinonediimine ligands and one phenanthroline ligand
Preparation Methods
Synthetic Routes and Reaction Conditions
The synthesis of Bis(9,10-phenanthrenequinonediimine)(phenanthroline)rhodium(III) typically involves the reaction of rhodium(III) chloride with 9,10-phenanthrenequinone and phenanthroline under controlled conditions. One common method includes the use of a titanium tetrachloride (TiCl4) and 1,4-diazabicyclo[2.2.2]octane (DABCO) system at elevated temperatures (around 140°C) to facilitate the formation of the desired product .
Industrial Production Methods
While specific industrial production methods for this compound are not extensively documented, the general approach would involve scaling up the laboratory synthesis procedures. This would include optimizing reaction conditions, ensuring the purity of reagents, and employing efficient purification techniques to obtain the compound in larger quantities.
Chemical Reactions Analysis
Types of Reactions
Bis(9,10-phenanthrenequinonediimine)(phenanthroline)rhodium(III) undergoes various types of chemical reactions, including:
Oxidation and Reduction: The compound can participate in redox reactions due to the redox-active nature of the 9,10-phenanthrenequinonediimine ligands.
Common Reagents and Conditions
Common reagents used in these reactions include oxidizing agents like hydrogen peroxide (H2O2) and reducing agents such as sodium borohydride (NaBH4). The reactions are typically carried out in solvents like acetonitrile or dichloromethane under controlled temperatures and inert atmosphere conditions .
Major Products Formed
The major products formed from these reactions depend on the specific reagents and conditions used. For example, oxidation reactions may yield oxidized forms of the ligands, while substitution reactions can result in new coordination complexes with different ligands .
Scientific Research Applications
Bis(9,10-phenanthrenequinonediimine)(phenanthroline)rhodium(III) has a wide range of scientific research applications:
Mechanism of Action
The mechanism by which Bis(9,10-phenanthrenequinonediimine)(phenanthroline)rhodium(III) exerts its effects involves its ability to intercalate into DNA and facilitate electron transfer processes. The rhodium(III) center plays a crucial role in mediating redox reactions, while the ligands stabilize the complex and enhance its binding affinity to nucleic acids . This interaction with DNA can lead to various biochemical effects, including the modulation of gene expression and the induction of oxidative stress .
Comparison with Similar Compounds
Similar Compounds
Bis(phenanthrenequinonediimine)(bipyridyl)rhodium(III): This compound features bipyridyl ligands instead of phenanthroline, resulting in different electronic and structural properties.
Bis(phenanthroline)(dipyridophenazine)ruthenium(II): A ruthenium-based analog that also intercalates into DNA and participates in electron transfer reactions.
Uniqueness
Bis(9,10-phenanthrenequinonediimine)(phenanthroline)rhodium(III) is unique due to its specific combination of ligands and the rhodium(III) center, which imparts distinct redox properties and a high affinity for DNA intercalation. This makes it particularly valuable in studies related to DNA-mediated electron transfer and its potential therapeutic applications .
Properties
Molecular Formula |
C38H26N6Rh-2 |
---|---|
Molecular Weight |
669.6 g/mol |
IUPAC Name |
(10-azanidylphenanthren-9-yl)azanide;1,10-phenanthroline;rhodium |
InChI |
InChI=1S/C14H10N2.2C12H8N2.Rh/c15-13-11-7-3-1-5-9(11)10-6-2-4-8-12(10)14(13)16;2*1-3-9-5-6-10-4-2-8-14-12(10)11(9)13-7-1;/h1-8,15-16H;2*1-8H;/q-2;;; |
InChI Key |
CJDVYSJAAAEVPW-UHFFFAOYSA-N |
Canonical SMILES |
C1=CC=C2C(=C1)C3=CC=CC=C3C(=C2[NH-])[NH-].C1=CC2=C(C3=C(C=CC=N3)C=C2)N=C1.C1=CC2=C(C3=C(C=CC=N3)C=C2)N=C1.[Rh] |
Origin of Product |
United States |
Disclaimer and Information on In-Vitro Research Products
Please be aware that all articles and product information presented on BenchChem are intended solely for informational purposes. The products available for purchase on BenchChem are specifically designed for in-vitro studies, which are conducted outside of living organisms. In-vitro studies, derived from the Latin term "in glass," involve experiments performed in controlled laboratory settings using cells or tissues. It is important to note that these products are not categorized as medicines or drugs, and they have not received approval from the FDA for the prevention, treatment, or cure of any medical condition, ailment, or disease. We must emphasize that any form of bodily introduction of these products into humans or animals is strictly prohibited by law. It is essential to adhere to these guidelines to ensure compliance with legal and ethical standards in research and experimentation.