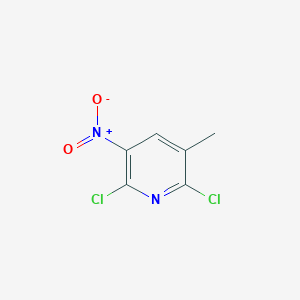
2,6-Dichloro-3-methyl-5-nitropyridine
Overview
Description
2,6-Dichloro-3-methyl-5-nitropyridine is a chemical compound with the molecular formula C6H4Cl2N2O2 and a molecular weight of 207.02 g/mol . It is a derivative of pyridine, characterized by the presence of two chlorine atoms, one methyl group, and one nitro group attached to the pyridine ring. This compound is known for its applications in various chemical reactions and its role as an intermediate in the synthesis of other complex molecules.
Mechanism of Action
Pharmacokinetics
Its physicochemical properties such as high density and low water solubility suggest that its bioavailability might be limited .
Result of Action
It’s known that nitropyridines can undergo macrocyclic condensation reaction with resorcinol derivatives to yield chiral tetraoxacalix arene pyridines .
Action Environment
It’s known that it should be stored in an inert atmosphere at room temperature , suggesting that it might be sensitive to oxygen and temperature changes.
Preparation Methods
Synthetic Routes and Reaction Conditions: The synthesis of 2,6-Dichloro-3-methyl-5-nitropyridine typically involves the nitration of 2,6-dichloro-3-methylpyridine. The nitration process is carried out using a mixture of concentrated nitric acid and sulfuric acid under controlled temperature conditions to ensure the selective introduction of the nitro group at the desired position on the pyridine ring .
Industrial Production Methods: Industrial production of this compound follows similar synthetic routes but on a larger scale. The process involves the use of large reactors and precise control of reaction parameters to achieve high yields and purity. The product is then purified through recrystallization or other suitable purification techniques to obtain the desired quality for industrial applications .
Chemical Reactions Analysis
Types of Reactions: 2,6-Dichloro-3-methyl-5-nitropyridine undergoes various chemical reactions, including:
Substitution Reactions: The chlorine atoms can be substituted by other nucleophiles such as amines, thiols, or alkoxides under appropriate conditions.
Reduction Reactions: The nitro group can be reduced to an amino group using reducing agents like hydrogen gas in the presence of a catalyst or other chemical reducing agents.
Coupling Reactions: It can participate in Suzuki-Miyaura coupling reactions with boronic acids to form carbon-carbon bonds.
Common Reagents and Conditions:
Substitution Reactions: Reagents such as sodium methoxide, potassium thiolate, or primary amines are commonly used. The reactions are typically carried out in polar aprotic solvents like dimethylformamide or dimethyl sulfoxide at elevated temperatures.
Reduction Reactions: Common reducing agents include palladium on carbon with hydrogen gas, or chemical reductants like tin(II) chloride in hydrochloric acid.
Coupling Reactions: Palladium catalysts and bases like potassium carbonate are used in the presence of boronic acids under inert atmosphere conditions.
Major Products Formed:
Substitution Reactions: Products include substituted pyridines with various functional groups replacing the chlorine atoms.
Reduction Reactions: The major product is 2,6-dichloro-3-methyl-5-aminopyridine.
Coupling Reactions: The products are biaryl compounds formed by the coupling of the pyridine ring with aromatic boronic acids.
Scientific Research Applications
2,6-Dichloro-3-methyl-5-nitropyridine has several applications in scientific research:
Chemistry: It is used as an intermediate in the synthesis of more complex organic molecules, including pharmaceuticals and agrochemicals.
Biology: The compound is studied for its potential biological activities, including antimicrobial and anticancer properties.
Medicine: It serves as a building block in the development of new therapeutic agents.
Industry: It is used in the production of dyes, pigments, and other specialty chemicals.
Comparison with Similar Compounds
- 2,6-Dichloro-3-nitropyridine
- 2,6-Dichloro-4-methoxy-3-nitropyridine
- 2,4,6-Trichloro-3-nitropyridine
- 2-Chloro-3-methyl-5-nitropyridine
Comparison: 2,6-Dichloro-3-methyl-5-nitropyridine is unique due to the presence of both methyl and nitro groups on the pyridine ring, which influences its reactivity and applications. Compared to 2,6-Dichloro-3-nitropyridine, the methyl group in this compound provides additional steric and electronic effects, making it more suitable for specific synthetic applications .
Properties
IUPAC Name |
2,6-dichloro-3-methyl-5-nitropyridine | |
---|---|---|
Source | PubChem | |
URL | https://pubchem.ncbi.nlm.nih.gov | |
Description | Data deposited in or computed by PubChem | |
InChI |
InChI=1S/C6H4Cl2N2O2/c1-3-2-4(10(11)12)6(8)9-5(3)7/h2H,1H3 | |
Source | PubChem | |
URL | https://pubchem.ncbi.nlm.nih.gov | |
Description | Data deposited in or computed by PubChem | |
InChI Key |
XLKDPPLSOTVMFR-UHFFFAOYSA-N | |
Source | PubChem | |
URL | https://pubchem.ncbi.nlm.nih.gov | |
Description | Data deposited in or computed by PubChem | |
Canonical SMILES |
CC1=CC(=C(N=C1Cl)Cl)[N+](=O)[O-] | |
Source | PubChem | |
URL | https://pubchem.ncbi.nlm.nih.gov | |
Description | Data deposited in or computed by PubChem | |
Molecular Formula |
C6H4Cl2N2O2 | |
Source | PubChem | |
URL | https://pubchem.ncbi.nlm.nih.gov | |
Description | Data deposited in or computed by PubChem | |
DSSTOX Substance ID |
DTXSID30483105 | |
Record name | 2,6-DICHLORO-3-METHYL-5-NITROPYRIDINE | |
Source | EPA DSSTox | |
URL | https://comptox.epa.gov/dashboard/DTXSID30483105 | |
Description | DSSTox provides a high quality public chemistry resource for supporting improved predictive toxicology. | |
Molecular Weight |
207.01 g/mol | |
Source | PubChem | |
URL | https://pubchem.ncbi.nlm.nih.gov | |
Description | Data deposited in or computed by PubChem | |
CAS No. |
58596-88-6 | |
Record name | 2,6-DICHLORO-3-METHYL-5-NITROPYRIDINE | |
Source | EPA DSSTox | |
URL | https://comptox.epa.gov/dashboard/DTXSID30483105 | |
Description | DSSTox provides a high quality public chemistry resource for supporting improved predictive toxicology. | |
Synthesis routes and methods
Procedure details
Disclaimer and Information on In-Vitro Research Products
Please be aware that all articles and product information presented on BenchChem are intended solely for informational purposes. The products available for purchase on BenchChem are specifically designed for in-vitro studies, which are conducted outside of living organisms. In-vitro studies, derived from the Latin term "in glass," involve experiments performed in controlled laboratory settings using cells or tissues. It is important to note that these products are not categorized as medicines or drugs, and they have not received approval from the FDA for the prevention, treatment, or cure of any medical condition, ailment, or disease. We must emphasize that any form of bodily introduction of these products into humans or animals is strictly prohibited by law. It is essential to adhere to these guidelines to ensure compliance with legal and ethical standards in research and experimentation.