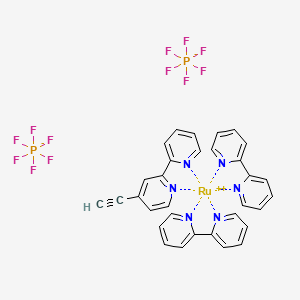
(2,2'-Bipyridine)(4-ethynyl-2,2'-bipyridine)Ruthenium hexafluorophosphate
- Click on QUICK INQUIRY to receive a quote from our team of experts.
- With the quality product at a COMPETITIVE price, you can focus more on your research.
Overview
Description
(2,2’-Bipyridine)(4-ethynyl-2,2’-bipyridine)Ruthenium hexafluorophosphate is a coordination compound that features a ruthenium center coordinated to two bipyridine ligands and one ethynyl-substituted bipyridine ligand. This compound is known for its unique electrochemical and photophysical properties, making it a subject of interest in various fields of scientific research.
Preparation Methods
Synthetic Routes and Reaction Conditions
The synthesis of (2,2’-Bipyridine)(4-ethynyl-2,2’-bipyridine)Ruthenium hexafluorophosphate typically involves the reaction of ruthenium precursors with bipyridine ligands. One common method involves the reaction of [Ru(bipy)2Cl2]·2H2O with 4-ethynyl-2,2’-bipyridine in the presence of a suitable base, followed by the addition of hexafluorophosphate anion to form the final product .
Industrial Production Methods
Industrial production methods for this compound are not well-documented, likely due to its specialized applications and the complexity of its synthesis. the general approach would involve scaling up the laboratory synthesis methods, ensuring precise control over reaction conditions to maintain product purity and yield.
Chemical Reactions Analysis
Types of Reactions
(2,2’-Bipyridine)(4-ethynyl-2,2’-bipyridine)Ruthenium hexafluorophosphate undergoes various types of chemical reactions, including:
Reduction: Reduction reactions can revert the oxidized ruthenium back to its lower oxidation states.
Substitution: Ligand substitution reactions can occur, where one or more ligands coordinated to the ruthenium center are replaced by other ligands.
Common Reagents and Conditions
Common reagents used in these reactions include oxidizing agents like cerium(IV) ammonium nitrate for oxidation, and reducing agents like sodium borohydride for reduction. Substitution reactions often require the presence of coordinating solvents or additional ligands .
Major Products Formed
The major products formed from these reactions depend on the specific conditions and reagents used. For example, oxidation reactions may yield ruthenium(III) complexes, while substitution reactions can produce a variety of ruthenium complexes with different ligand environments .
Scientific Research Applications
(2,2’-Bipyridine)(4-ethynyl-2,2’-bipyridine)Ruthenium hexafluorophosphate has a wide range of scientific research applications, including:
Mechanism of Action
The mechanism of action of (2,2’-Bipyridine)(4-ethynyl-2,2’-bipyridine)Ruthenium hexafluorophosphate involves its ability to undergo metal-to-ligand charge transfer (MLCT) transitions. These transitions result in the generation of excited states that can participate in various photochemical and electrochemical processes. The compound’s molecular targets and pathways include interactions with DNA, proteins, and other biomolecules, leading to its applications in bioimaging and therapeutic research .
Comparison with Similar Compounds
Similar Compounds
Tris(2,2’-bipyridine)ruthenium(II) hexafluorophosphate: Known for its use in electrochemical and photochemical applications.
(4,4’-Bisphenyl-ethynyl-2,2’-bipyridyl)Rhenium tricarbonyl chloride: Used as a catalyst for CO2 reduction.
Uniqueness
(2,2’-Bipyridine)(4-ethynyl-2,2’-bipyridine)Ruthenium hexafluorophosphate is unique due to its combination of bipyridine and ethynyl-substituted bipyridine ligands, which confer distinct electrochemical and photophysical properties. This makes it particularly suitable for applications requiring precise control over electronic and photonic behavior .
Biological Activity
The compound (2,2'-Bipyridine)(4-ethynyl-2,2'-bipyridine)ruthenium hexafluorophosphate is a ruthenium complex that has garnered attention for its potential biological applications, particularly in the fields of photodynamic therapy (PDT) and anticancer treatments. Ruthenium complexes are known for their unique properties, including the ability to generate reactive oxygen species (ROS), which can induce cytotoxic effects in cancer cells. This article reviews the biological activity of this compound, focusing on its synthesis, characterization, and biological investigations.
Synthesis and Characterization
The synthesis of this compound typically involves the coordination of bipyridine ligands to a ruthenium center. The resulting complex is characterized by various spectroscopic techniques including:
- Nuclear Magnetic Resonance (NMR) : Used to determine the structure and purity of the synthesized compound.
- Infrared Spectroscopy (IR) : Helps identify functional groups and confirm ligand coordination.
- Ultraviolet-Visible Spectroscopy (UV-Vis) : Assesses electronic transitions and stability in solution.
- X-ray Crystallography : Provides detailed structural information about the arrangement of atoms in the crystal lattice.
Cytotoxicity
Research has demonstrated that ruthenium complexes exhibit significant cytotoxicity against various cancer cell lines. For instance, studies have shown that complexes containing 2,2'-bipyridine ligands can effectively induce apoptosis in human cancer cells through ROS generation upon light irradiation . The cytotoxic effects are often evaluated using assays such as:
- MTT Assay : Measures cell viability post-treatment.
- Annexin V/PI Staining : Assesses apoptosis levels in treated cells.
Anticancer Mechanisms
The mechanisms by which these complexes exert their anticancer effects include:
- DNA Binding : Ruthenium complexes can intercalate into DNA, leading to structural alterations that trigger cellular stress responses .
- Reactive Oxygen Species Generation : Upon activation by light, these complexes can produce ROS, which damage cellular components and induce apoptosis .
- Protein Interactions : Binding to serum proteins like BSA (bovine serum albumin) can enhance the bioavailability of the drug and facilitate its delivery to target cells .
Study 1: Photodynamic Therapy Applications
A study investigated the use of a related ruthenium complex in PDT. The results indicated that upon irradiation with visible light, the complex generated ROS that significantly inhibited the growth of MCF-7 breast cancer cells. The authors concluded that the compound's ability to produce ROS upon light activation makes it a promising candidate for PDT applications .
Study 2: Anticancer Efficacy
Another research effort focused on a series of bipyridine-ruthenium complexes with varying substituents. The study reported that certain derivatives exhibited potent antiproliferative activity against U-118MG glioblastoma cells. The mechanism was attributed to their ability to bind DNA and generate oxidative stress within the cells .
Data Tables
Compound | Cell Line Tested | IC50 Value (µM) | Mechanism |
---|---|---|---|
Complex A | MCF-7 | 15 | ROS Generation |
Complex B | U-118MG | 10 | DNA Binding |
Complex C | L6 | 20 | Apoptosis Induction |
Properties
Molecular Formula |
C32H24F12N6P2Ru |
---|---|
Molecular Weight |
883.6 g/mol |
IUPAC Name |
4-ethynyl-2-pyridin-2-ylpyridine;2-pyridin-2-ylpyridine;ruthenium(2+);dihexafluorophosphate |
InChI |
InChI=1S/C12H8N2.2C10H8N2.2F6P.Ru/c1-2-10-6-8-14-12(9-10)11-5-3-4-7-13-11;2*1-3-7-11-9(5-1)10-6-2-4-8-12-10;2*1-7(2,3,4,5)6;/h1,3-9H;2*1-8H;;;/q;;;2*-1;+2 |
InChI Key |
USBRJFBKNBEQBS-UHFFFAOYSA-N |
Canonical SMILES |
C#CC1=CC(=NC=C1)C2=CC=CC=N2.C1=CC=NC(=C1)C2=CC=CC=N2.C1=CC=NC(=C1)C2=CC=CC=N2.F[P-](F)(F)(F)(F)F.F[P-](F)(F)(F)(F)F.[Ru+2] |
Origin of Product |
United States |
Disclaimer and Information on In-Vitro Research Products
Please be aware that all articles and product information presented on BenchChem are intended solely for informational purposes. The products available for purchase on BenchChem are specifically designed for in-vitro studies, which are conducted outside of living organisms. In-vitro studies, derived from the Latin term "in glass," involve experiments performed in controlled laboratory settings using cells or tissues. It is important to note that these products are not categorized as medicines or drugs, and they have not received approval from the FDA for the prevention, treatment, or cure of any medical condition, ailment, or disease. We must emphasize that any form of bodily introduction of these products into humans or animals is strictly prohibited by law. It is essential to adhere to these guidelines to ensure compliance with legal and ethical standards in research and experimentation.